









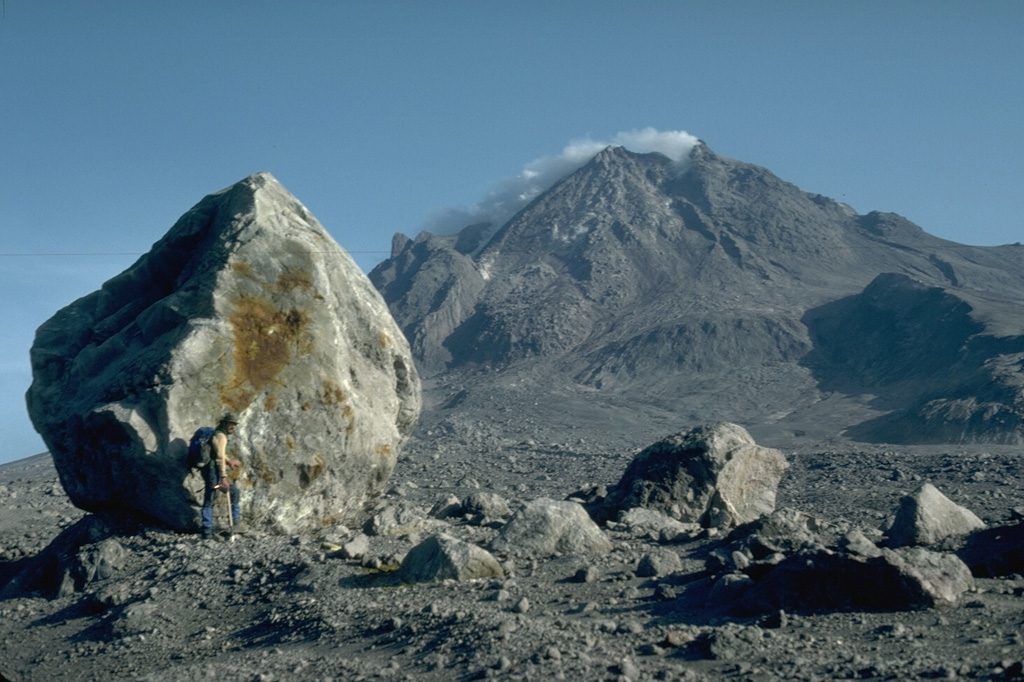
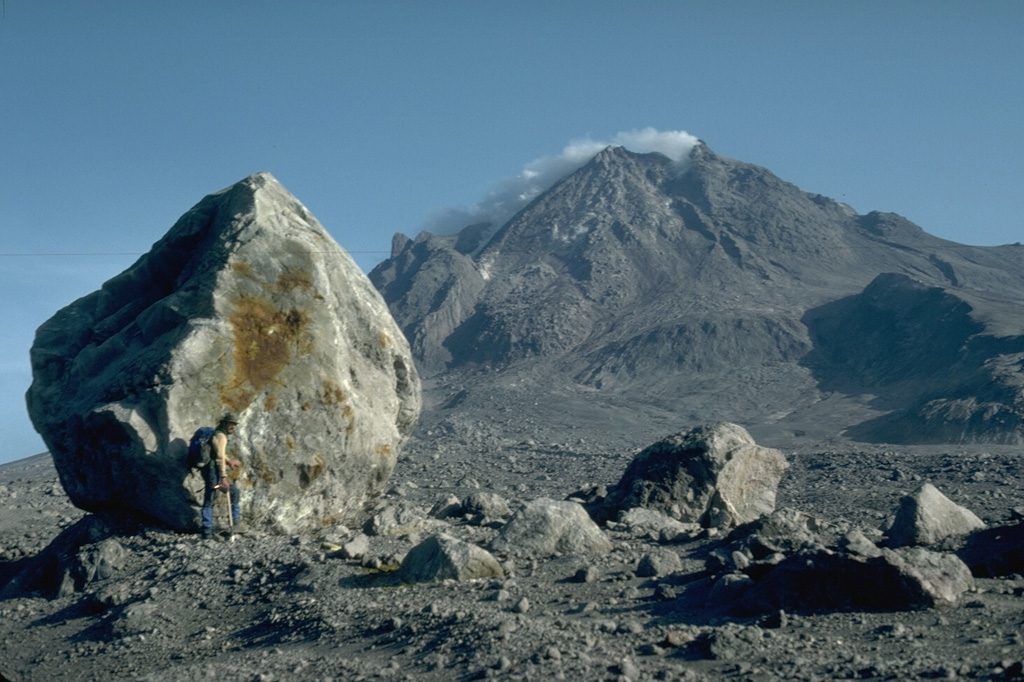
This 6 m high lava block was carried 4 km down the flank of Augustine volcano (Alaska) by a block and ash flow during the 1976 eruption. Image Credit: Harry Glicken, 1986 (U.S. Geological Survey/Smithsonian Inst.)
Block and ash flows – a hot pyroclastic flows that transport big chunks of rock
Block and ash flows (BAFs) are distinguished from other pyroclastic density currents (PDC) by their coarse blocky textures and derivation from the collapse of lava domes. Like any PDC, they are fast moving, hot, and capable of wrecking widespread death and destruction. Well known historical examples of eruptions that produced devastating PDCs following lava dome collapse include Soufriere (1902, 1979), Mount St. Helens (1981), Merapi (Indonesia,2006), and Unzen (Japan, 1990-95).
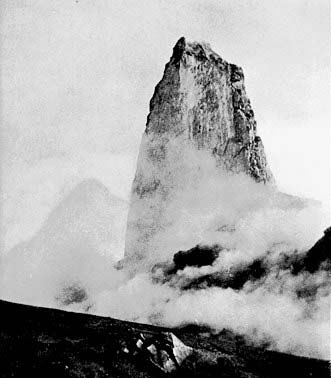
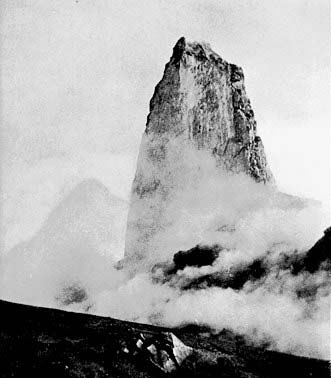
The Mt Pelée spine began growing October 8, 1902, about 4 months after the catastrophic nuée ardent that destroyed the town of Saint-Pierre. Growing up to 15 m per day, it reached a height of 300 m. Collapse of this spine also generated block and ash flows. Image credit: Angelo Heilprin, 1902, one of the first geologists on the scene after the eruption.
Diagnostic field and outcrop characteristics derived from observations of recent PDCs and ancient deposits include:
- Like most PDCs, a BAF consists of a lower, concentrated blocky ash flow overlain by a more dilute, billowing (turbulent), surging ash plume that deposits finer grained, commonly stratified ash and lapilli.
- Flow speeds up to 30 m/sec are common (~100 km/hr); slower than ignimbrites, but still difficult to outrun!
- Fragments are usually dense, poorly vesicular lava, rather than the highly vesicular pumices characteristic of ignimbrites.
- Fragments commonly show flow banding and vesicle alignment inherited from the parent lava dome.
- Compositions range from andesite to rhyolite (the compositions of the parent lava domes), tending towards the more felsic end of that spectrum.
- They are hot (up to 600o C) but tend to be non-welded. Entrained vegetation is incinerated or carbonised.
- Their distribution is governed primarily by topography, thickest in valleys (10s of m thick), but potentially spilling over adjacent interfluves. Most recent BAFs have runout distances of a few kilometres down the flanks of the volcanic edifice from which they originated (e.g., Merapi, Unzen). One notable exception has been observed in recent flows on Shiveluch volcano (2005, 2010, Kamchatka) where blocks 5 m in diameter were transported 15 km from the lava dome ( Krippner et al., Characterizing the 2005 and 2010 giant block-and-ash flow deposits at Shiveluch using high-resolution satellite and field data. In press, Volcanica).
- They are poorly sorted, and either non-graded or show some degree of normal or reverse coarse-tail grading.
- Closely spaced stratigraphic sections may show significant variability in thickness, grain size distribution and grading, and stratification.
- BAFs are commonly associated with pyroclastic surges.
- BAFs are likely to be interstratified with airfall deposits and large ballistics derived from explosive eruptions, other PDCs derived from collapsing plinian and vulcanian eruption columns, and lahars deposited in intervals between eruptive episodes.
Lava domes
Block and ash flows are mostly derived from the collapse of lava domes. Compared to PDCs, the growth of lava domes is a relatively quiet affair, in craters or adjacent flanks of volcanoes at the outlet of active vents. They tend to form from viscous lava at the felsic end of the compositional spectrum, although variations in viscosity will determine the manner in which magma is extruded. Two basic styles of extrusion and dome growth are recognized:
BAF deposits consist of angular to subrounded juvenile fragments ranging from lapilli to blocks exceeding 10 m across, set in a finer ash matrix. The proportion of matrix is variable – those BAFs with minimal matrix are referred to as fines-poor or fines-depleted.
- Endogenic domes that expand as magma is intruded into the dome interior (i.e., they inflate from within), and
- Exogenic domes that grow externally by addition and stacking of lava flows.
There are variations between these two end-members and one style of extrusion may evolve into the other depending on changes in magma composition, viscosity, and extrusion rate (Fink and Anderson, 2000). Dome shapes range from symmetrical, hemispherical structures, to steep-sided spines. Growth of lava domes is accompanied by development of flow banding, shear zones, and fractures indicating that flow rheology can involve both ductile and brittle behaviour. The outer carapace of cooled lava is characteristically fractured during cooling and dome expansion (extensional fractures), leading to an almost continuous supply of angular fragments accumulating in talus fans around the dome base (these are mostly cold deposits). Talus debris can be incorporated into PDCs or redeposited as lahars.


The Mount St. Helen dome in, 1984 (left) continued to grow until 1986 – at this point it was 1.1 km wide and 250 m high. Image credit: Lyn Topinka, 1984 (U.S. Geological Survey/Smithsonian Inst.). Right: Mount St. Helen 2005 spire-like extrusion where fracturing and spalling provide debris for talus aprons. Image credit: U.S. Geological Survey, Cascades Volcano Observatory/Smithsonian Inst.).
Lava domes are inherently unstable. Dome collapse is driven by gravity acting on fractured, oversteepened slopes, or explosively from internal pressures. Gravitational slope failure may also lead to explosive behaviour – the major eruption at Mount St. Helens in 1981 was caused by the concatenation of such processes – a bit like popping the cork from a bottle of soda water (or champagne). Dome collapse is caused by (Ashwell et al. 2018):
- Gravitational instability of oversteepened, fractured dome surfaces.
- Undercutting of the dome base by slumping.
- Oversteepening caused by rapid increases in extrusion producing inflation or bulging.
- High precipitation.
Collapse of an actively growing dome will release hot rock and volatiles – it is these materials that produce the pyroclastic density currents – primarily block and ash flows and pyroclastic surges.
BAF flow mechanisms
BAFs are generally considered to act as concentrated flows of blocks and finer ash. However, unlike cold (non-volcanogenic) debris flows there is probably minimal matrix strength to support the flow. Instead, BAFs are often compared with grain flows where flow is maintained by dispersive pressures. This mechanism was first discovered by R.A. Bagnold from a combination of theory and experiments with moving sediment – fluid mixtures. Dispersive pressures derive from interparticle collisions in a fluid, and these collisions transfer momentum from one fragment to another, but in the process lose some kinetic energy. Reverse (or inverse) grading is a common manifestation of this process in grain flows and some debris flows, including lahars; this is the case where large fragments move to the top of the flow because they experience greater dispersive pressures than smaller fragments. Some BAFs also exhibit normal grain size grading or normal coarse-tail grading which suggests deposition from a waning turbulent flow. Thus, it is possible that BAFs, like other kinds of sediment density currents, undergo flow transitions as the flow evolves.
Observations of recent BAFs indicate that pyroclastic surges frequently develop as the overlying turbulent plume decouples from the main, concentrated flow body Hanenkamp, 2011; Krippner et al, in press). Pyroclastic surges tend to be much more dilute, fast-moving flows that progress from the front of BAFs or as overbank flows across channel-valley margins. Their deposits commonly are stratified, including bedforms manifested in outcrop as low amplitude crossbeds. Decoupling of the surges is initiated by elutriation of fine ash from the BAF, ingestion of air into the bulk flow, or diversion of the overlying ash cloud at channel bends and other topographic obstacles.
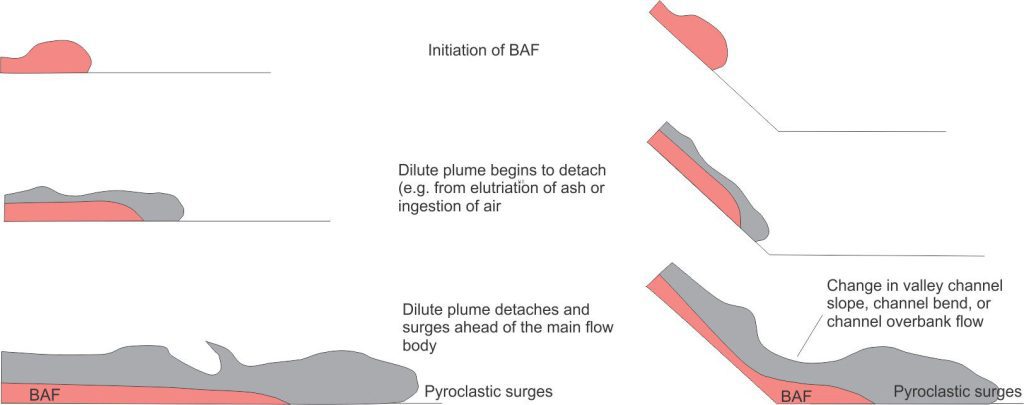
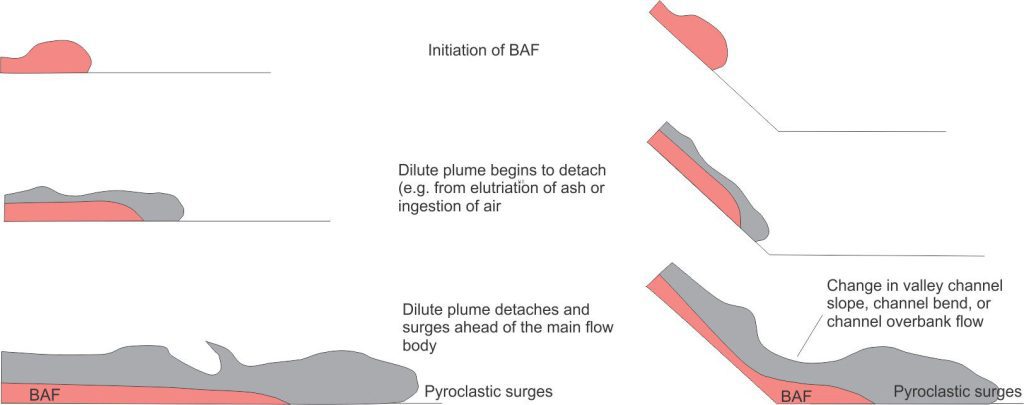
Evolution of a dilute, turbulent ash plume decoupling from a cascading block and ash flow (pink), along relatively flat ground (left), and where there is a change in depositional slope or topographic obstacle (right). Modified from E. Hanenkamp, 2011, Fig. 2.3, Canterbury University PhD thesis.
Block and ash flow deposits in outcrop
The images that follow are from the Okataina eruption centre southeast of Rotorua, New Zealand. The centre and its volcanic and volcaniclastic deposits are part of the Taupo Volcanic Zone. Violent supereruptions resulting in Okataina caldera collapse and resurgence began about 400 ka. The last 20,000 years have been particularly active, with 40 vents identified, including the youngest major eruption at Tarawera in 1886 (Nairn). The Okataina eruption centre is one of several caldera complexes in the Taupo Volcanic Zone that includes the iconic Taupo and Rotorua calderas.
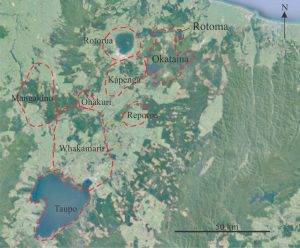
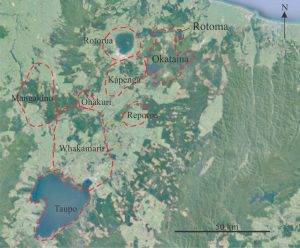
Approximate boundaries of major calderas in the active Taupo Volcanic Zone, New Zealand. Okataina eruption centre and Lake Rotoma are located in the northeast corner. Base map from Google Earth. Caldera boundaries from Leonard et al. 2010.
Rhyolitic and dacitic lava, pyroclastics, and airfall deposits are exposed in road cuts and along the shore of Lake Rotoma, formed about 7000-9000 years ago. The lavas are flow banded and contain abundant spherulitic obsidians. Pyroclastics include partly welded and non-welded ignimbrites, and block and ash flow deposits; at one locality the BAFs are sandwiched between flow banded rhyolite. Lava flows and PDCs are also draped by airfall deposits.


A block and ash flow derived from dome collapse at Mt. Tarawera. Note the angularity of blocks and coarse lapilli (to 50 cm wide in this view), extremely poor sorting, and lack of preferred clast alignment. The stratified ash beneath the BAF may be a surge deposit. Hammer lower centre. Image credit: Tarawera, Kari Cooper, 2017, National Science Foundation, public domain.


Left: Flow banded rhyolite and black obsidians stratigraphically associated with the BAF deposits shown in the image below. Right: Black spherulitic obsidian. The spherulites are spheroidal to oblate and consistently 2-3 mm in diameter. Both lithologies comprise the dominant fragmental rock types in the associated BAFs. Coin diameter is 22 mm. Southwest shore of Lake Rotoma, New Zealand.
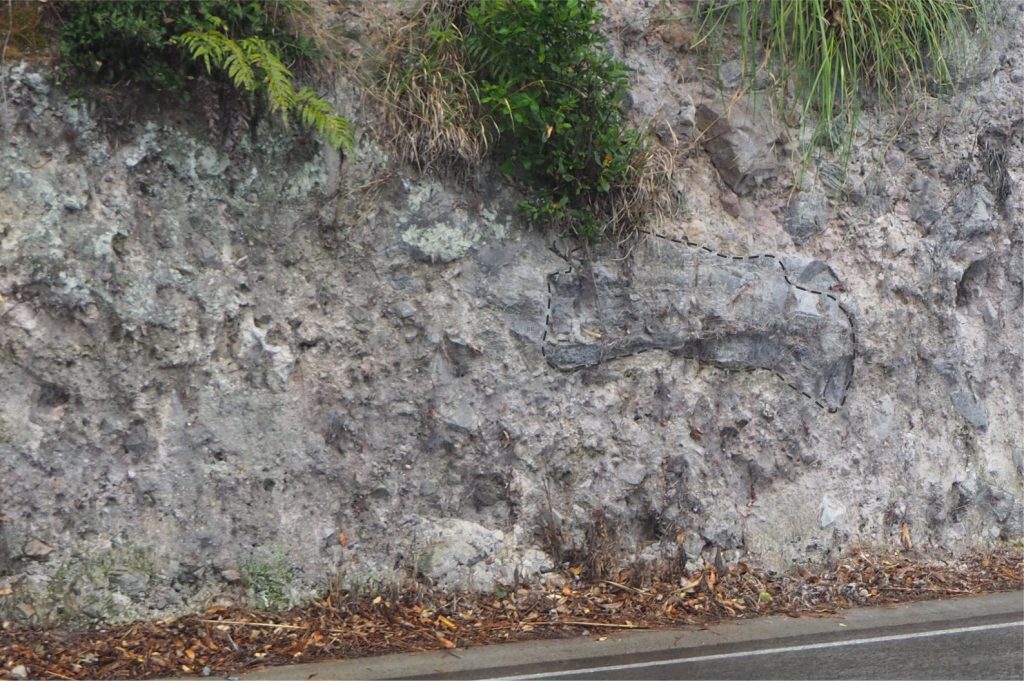
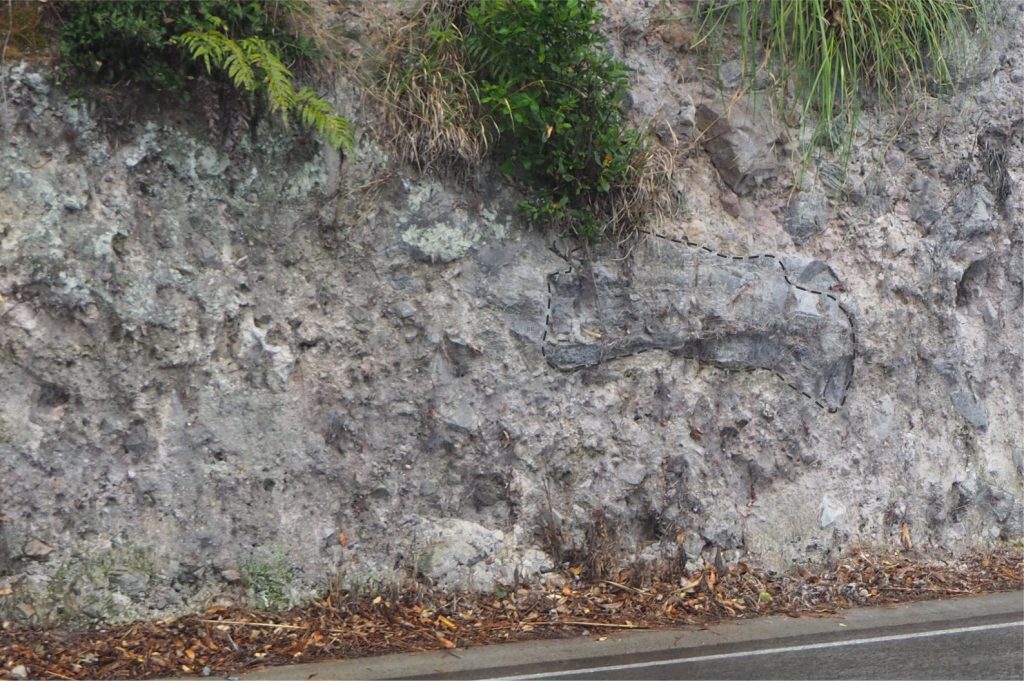
Block and ash flow deposit containing blocks and lapilli of dense rhyolite and flow banded obsidian. Note the 1.7 m long block at right centre (outlined). The deposit is very poorly sorted and lacks stratification or clast-size grading. Southwest shore of Lake Rotoma, New Zealand.
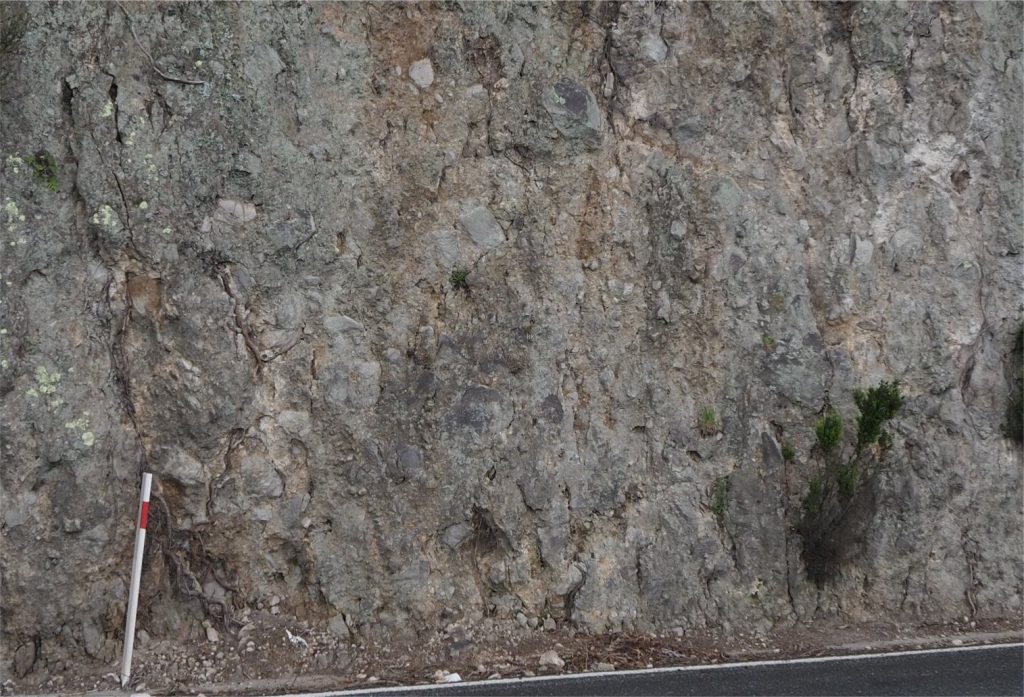
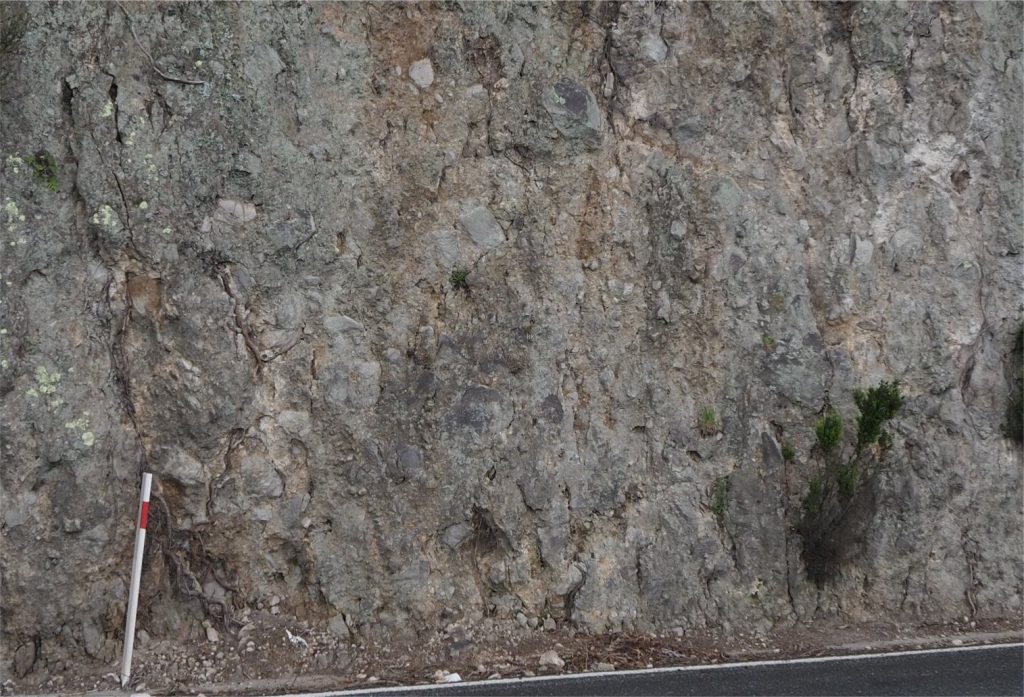
An example of a fines-depleted BAF containing a chaotic assortment of blocks ranging from lapilli to about a metre diameter. Sorting is extremely poor; blocks are angular. The framework is mostly clast-supported. There is no preferred alignment of clasts. Block compositions include abundant flow banded rhyolite and obsidian. Detailed views are shown in the two images below. The road marker is 1 m long. South shore of Lake Rotoma.
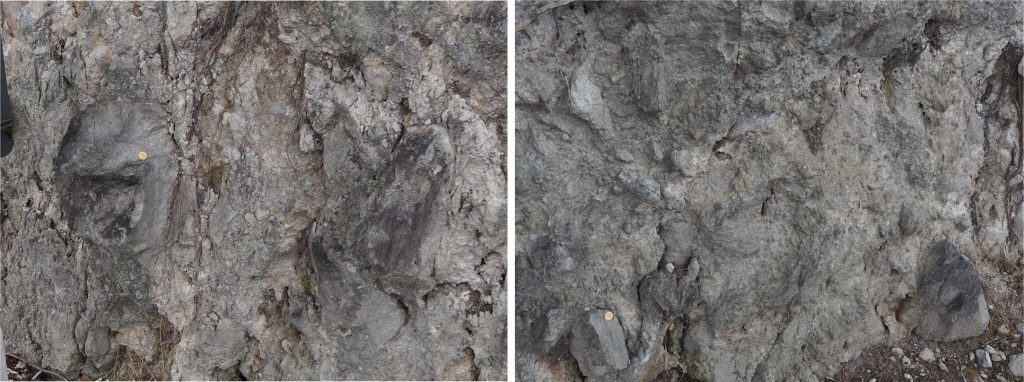
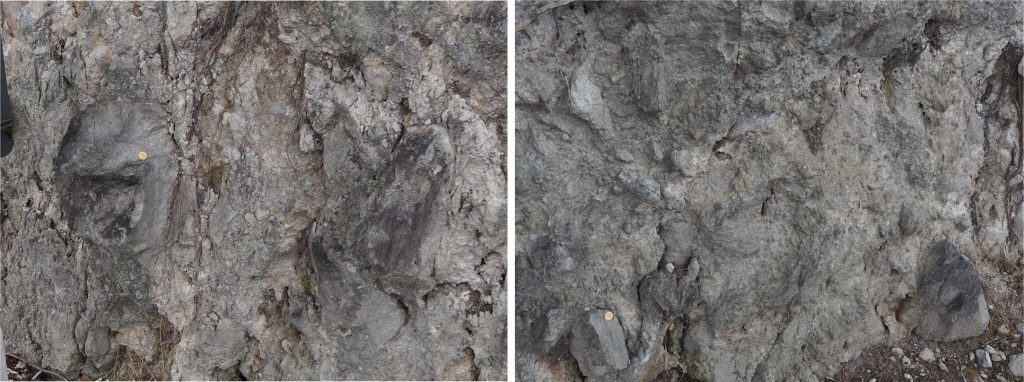
A closer look at the previous BAF example, emphasizing the clast-supported textures. Flow banding is visible in some obsidian and rhyolite blocks. This outcrop also contains significant fracturing – possibly caused by local tectonism during various eruptions, and/or by differential compaction from overlying flows and pyroclastics. Coin is 22 mm diameter. South shore of Lake Rotoma.
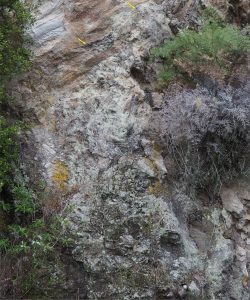
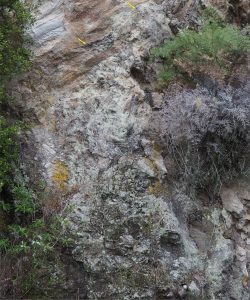
Another example of a fines poor BAF, this one sandwiched between flow banded rhyolites – that represent either lava flows or segments of lava domes. The base of the overlying flow has incorporated some blocks and sediment from the BAF (arrows). Outcrop is about 5 m high. Southeast shore of Lake Rotoma.
Related posts
Accretionary aggregates and accretionary lapilli
Ignimbrites in outcrop and thin section
Volcanics in outcrop: Pyroclastic density currents
Volcanics in outcrop: Secondary volcaniclastics
Volcanics in outcrop: Lava flows
Volcanics in outcrop: Pyroclastic fall deposits
Fluid flow: Froude and Reynolds numbers
Sediment transport: Bedload and suspension load
The hydraulics of sedimentation: Flow regime

















