









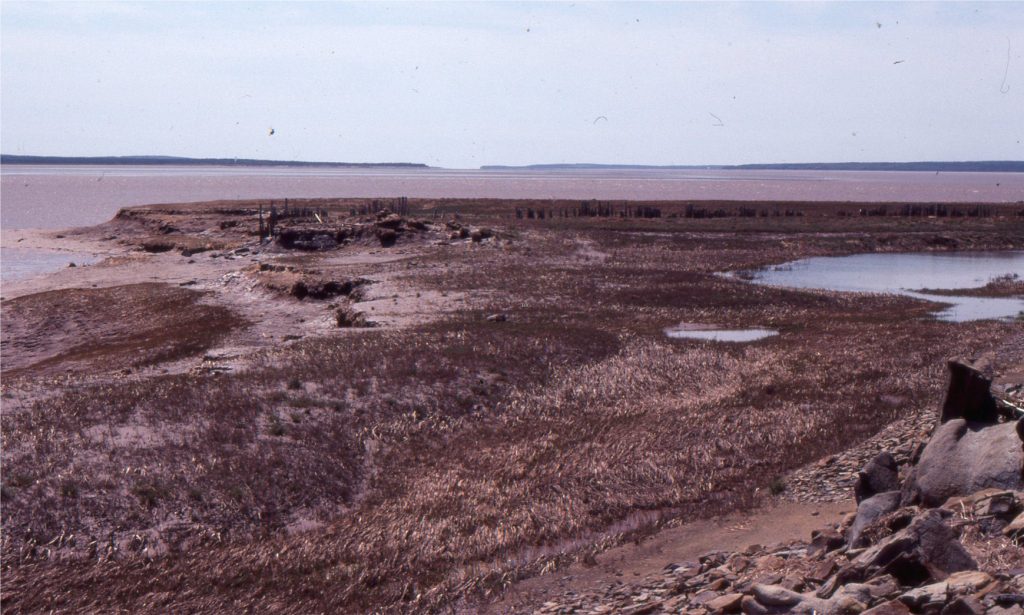
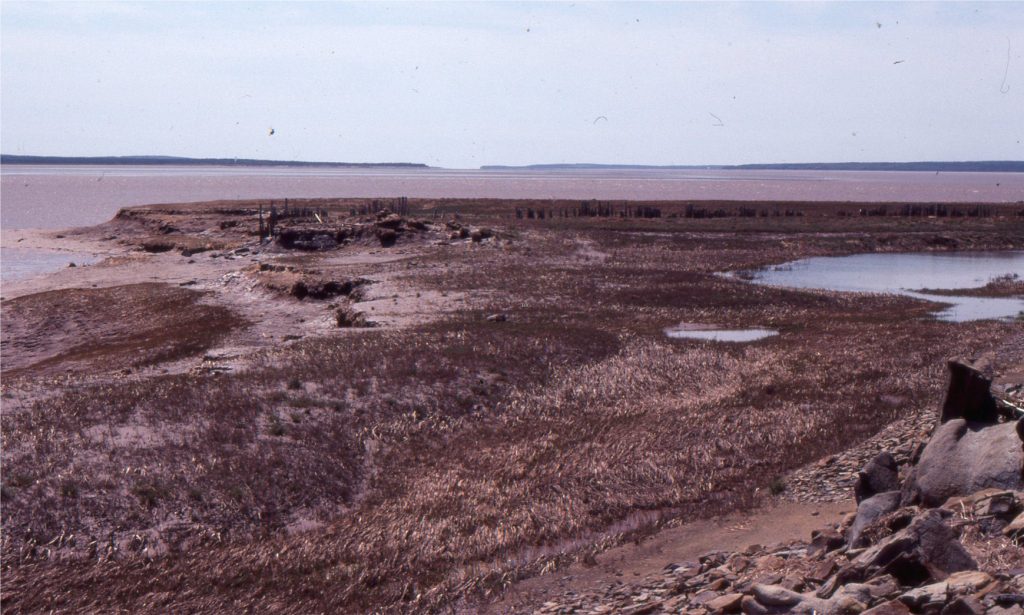
Several generations of salt marsh have formed along Fundy Bay coasts during the Holocene post-glacial, eustatic rise in sea level. At this location the seaward edge is bound by marsh cliffs that define at least three platforms at different elevations, each representing marsh development at different stages of sea level rise. Vegetation here is dominated by Spartina. Small, shallow pannes, or ponds (centre right) are recharged during precipitation and spring tide flooding.
This is the fourth post in a series on vegetated coastal lithofacies – see also:
Seagrass meadows and ecosystems
Seagrass lithofacies in the rock record
Salt marshes, along with mangrove and seagrass communities, occupy a unique position along many coasts, at the transition from intertidal to terrestrial environments. All three ecosystems also occupy a unique position in the stratigraphic convergence of marine and non-marine lithofacies. The locus of each ecosystem is generally defined as:
- Seagrasses occupy intertidal to shallow subtidal zones where there is daily or permanent submergence by tides.
- Mangroves thrive in the upper intertidal zone that is regularly flooded by tides, but where the vegetation is not submerged.
- Salt marshes occupy a more landward position where they are flooded, but not necessarily submerged, only during spring tides and storm surges.
Modern mangrove and seagrass ecosystems are restricted to tropical – subtropical-temperate latitudes, a situation that appears to have persisted in the rock record. In comparison, modern salt marshes are widely distributed globally, as far north as Greenland and Iceland and south to the Sub-Antarctic Auckland and Campbell islands. Thus, the salt marsh ecosystem may have greater paleogeographic and stratigraphic value.
Salt marsh ecosystems
Like mangrove ecosystems, salt marshes accumulate in low-energy estuaries, embayments and lagoons, and wetland areas behind beaches (the beaches themselves may be high energy, wave dominated but the back-beach area is sheltered). They occupy supratidal zones where seawater flooding only occurs during spring tides and storm surges. Soils are saline, but the degree of saturation alternates from fully saturated during tidal floods, to partly drained and even desiccated during exposure (Allen, 2000). There is little competition from mangroves under these conditions, but at the landward limits of the marshes there is increasing competition from non-halophytic coastal flora.
The most obvious characteristic of salt marshes is their flora, dominated by salt-tolerant (halophytic) plants like Spartina (cord grass) and Juncus, and succulents such as Salicornia. The variation and extent of different plant species depend on temperature and precipitation, the frequency of tidal inundation that also is related to marsh elevation and distance from tidal channels, the degree of desiccation, and soil drainage (Townend et al., 2010, PDF).
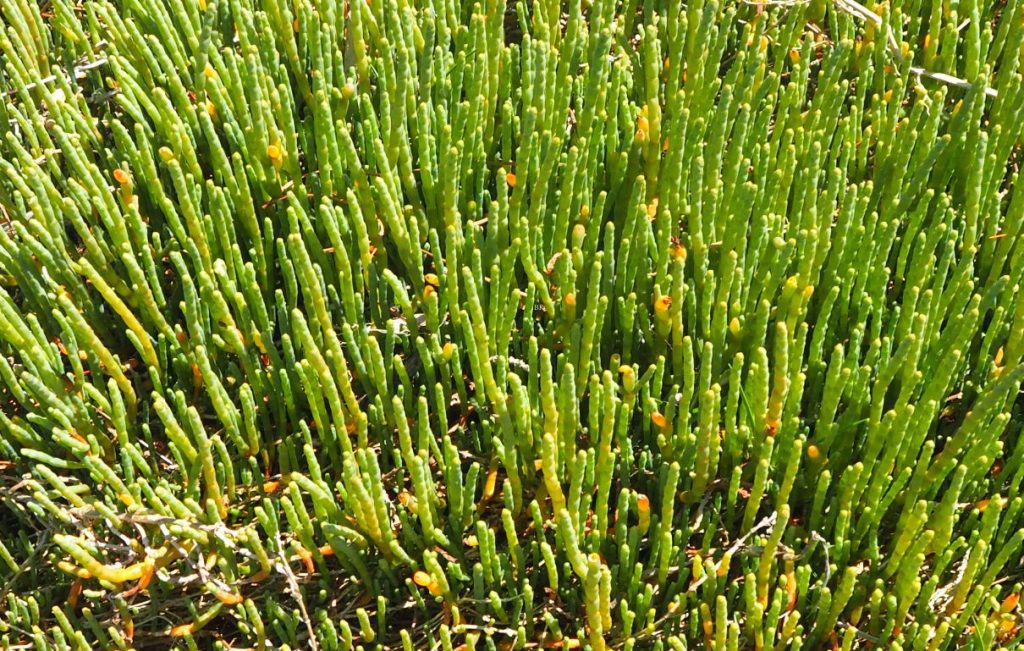
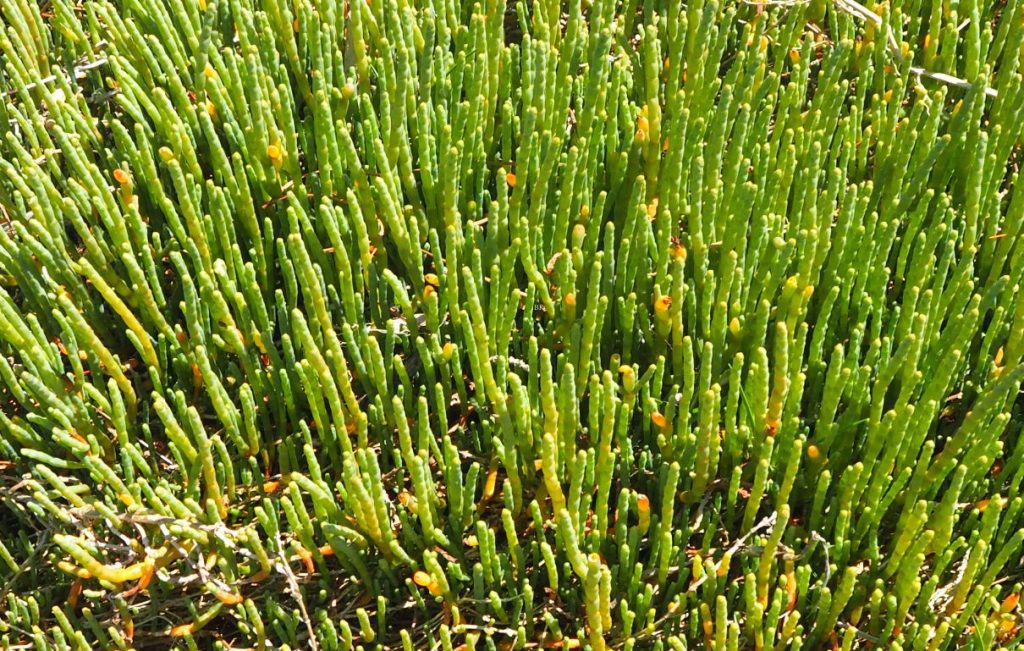
Salicornia is a common salt marsh inhabitant. Succulent plants like these are salt tolerant and well adapted to extended periods of soil drying. They also develop dense root tangles that help stabilise the marsh soils.
Sediment infaunal activity can be intense but is restricted to invertebrate species that can tolerate variable salinity and relatively long periods of exposure and potential desiccation. Worms and crabs are most common. Rampant bioturbation by these critters can have a major impact on soil drainage. Foraging birds are common; at some locations grazing by four-legged critters (particularly cattle) can seriously damage the flora and soil structure.
Salt marsh sedimentation
Salt marshes are flat, seaward-dipping platforms that either merge ramp-like with tidal flats or are in abrupt contact defined by micro-cliffs. The marshes are usually transected by branching tidal creek networks that tend to merge seaward to a single waterway. The channels are filled during normal flood tides, but only overtop during spring tides. Channel extent and depth is strongly correlated with tidal range and the magnitude of the tidal prism (the volume of seawater exchanged during a tidal cycle).
Small cliff-like structures are a common feature along the seaward margins of salt marshes. Most are centimetres to decimetres high. They are erosional structures usually associated with marsh retreat.
Allen (2000, op cit.) identified two main types of salt marsh based on sediment composition: organic-dominated and mineral-dominated. Organic marshes derive sediment primarily from local halophytic vegetation; accretion rates in this case are relatively low. Organic matter can accumulate during and between flood events, but it may be subject to aerobic or anaerobic degradation.
Mineralogical marshes derive sediment from the adjacent estuary or lagoon, consisting mostly of mud and silt that is carried in suspension by channel overbank flow; coarser-grained sediment may be introduced to the platform during storm surges. Local ponds, or pannes, dot the marsh surface and may harbour additional invertebrates because of extended periods of inundation. Panne sediment may contain high concentrations of organic matter, including that derived from macroalgae. Prolonged exposure of marshes and pannes can produce a variety of desiccation structures including mud cracks and curled or disrupted microbial mats.
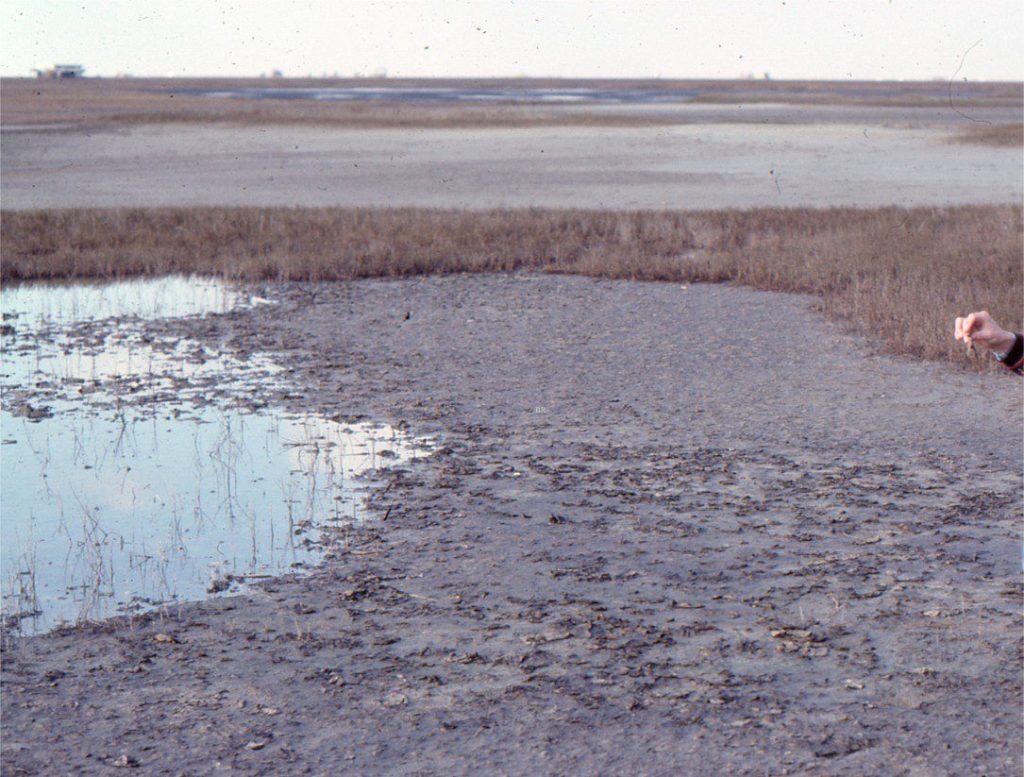
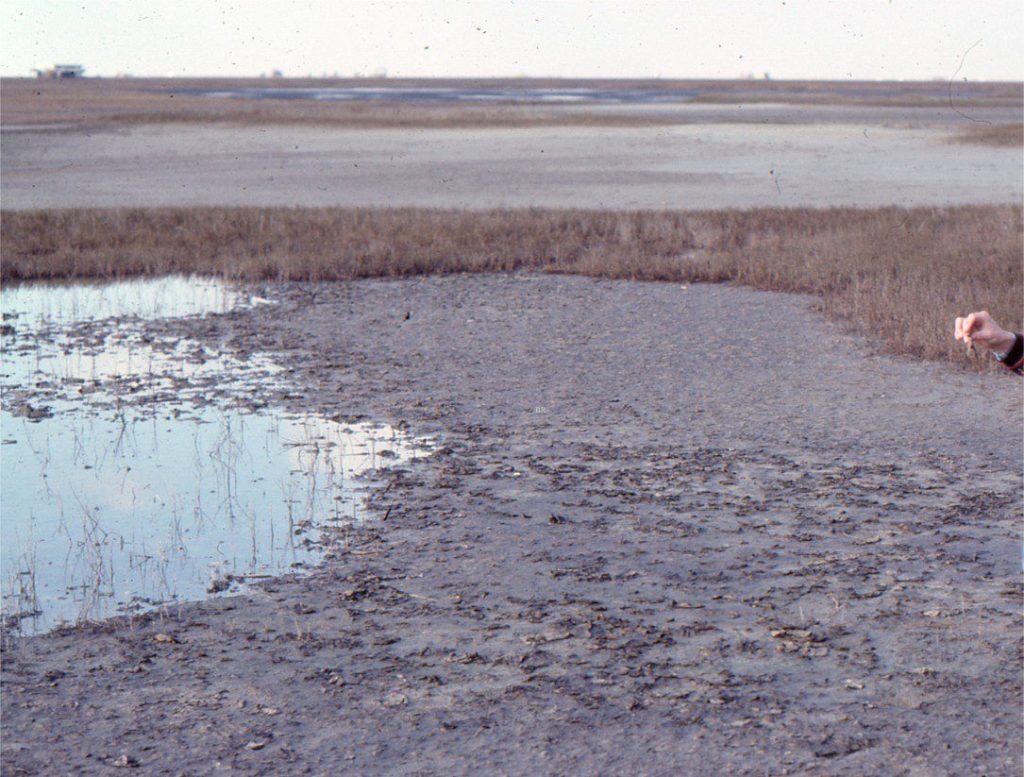
An extensive salt marsh has formed behind coastal dunes near Freeport, Texas. The swaths of Spartina are separated by supratidal, mixed sand-mud flats that are covered by microbial mats in various states of desiccation.
Mineralogical accretion of a marsh only occurs during spring tide inundation. Accretion rate and thickness depend on:
- The local accommodation space.
- The suspended sediment load concentration.
- Proximity to tidal channels and creeks,
- The length of time the platform surface is inundated – commonly referred to as the hydroperiod, and,
- The settling rate of suspended sediment.
Sedimentation on modern salt marshes is strongly influenced by local vegetation; tidal flow velocities are attenuated by the tangle of plants that trap sediment. Root tangles help bind the sediment. For net accretion to occur, the sedimentation rate during flood tides must exceed erosional losses during the subsequent ebb tides. Salt marsh vegetation can also attenuate storm waves.
Accommodation space
The importance of spring-tide flooding to salt marsh viability means that these ecosystems are strongly influenced by even subtle changes in relative sea level – specifically the dynamic relationship between changes in accommodation space and sediment supply. Such changes may be autocyclic, for example resulting from variations in local sediment supply, local tidal amplification, changes in the tidal channel network, or autocompaction of marsh sediment. Salt marsh platforms will also respond to allocyclic processes such as glacio-eustatic forcing of sea level rise and fall, or basin-wide tectonic subsidence or uplift.
Salt marsh survival based on its response to sea level rise is analogous to that commonly applied to the fate of coral reefs:
- Marsh accretion keeps up with sea level rise and is in equilibrium with the creation of accommodation space. Accretion in this context is based primarily on sedimentation rate, but also means that halophytic vegetation must be able to regenerate to maintain the essential character of the salt marsh.
- If sea level rise accelerates the marsh will need to catch up. This implies a time lag for sedimentation and regrowth to adjust to the new base level. Recent numerical modelling suggests that this lag may be a few decades (Kirwan and Temmerman, 2009, PDF).
- If it is unable to catch up, vegetation will not survive and the marsh will give up.
If sea level rise exceeds the sediment supply rate, then marsh accretion will not keep up. The marshes will eventually drown and, depending on the extent of transgression, may be subjected to ravinement as the shoreline and shoreface shifts landward. Shoreface ravinement has the potential for removal of some or all the salt marsh and associated paralic deposits; the deeper parts of tidal creeks may survive these erosional episodes.
If sediment supply exceeds the rate at which accommodation space is created (i.e., the rate of sea level rise is low, at still-stand, or decreasing), then the marsh will probably expand seaward in concert with the progradation of laterally associated coastal depositional systems (such as mangrove wetlands, lagoon, barrier island, beach, and shoreface).
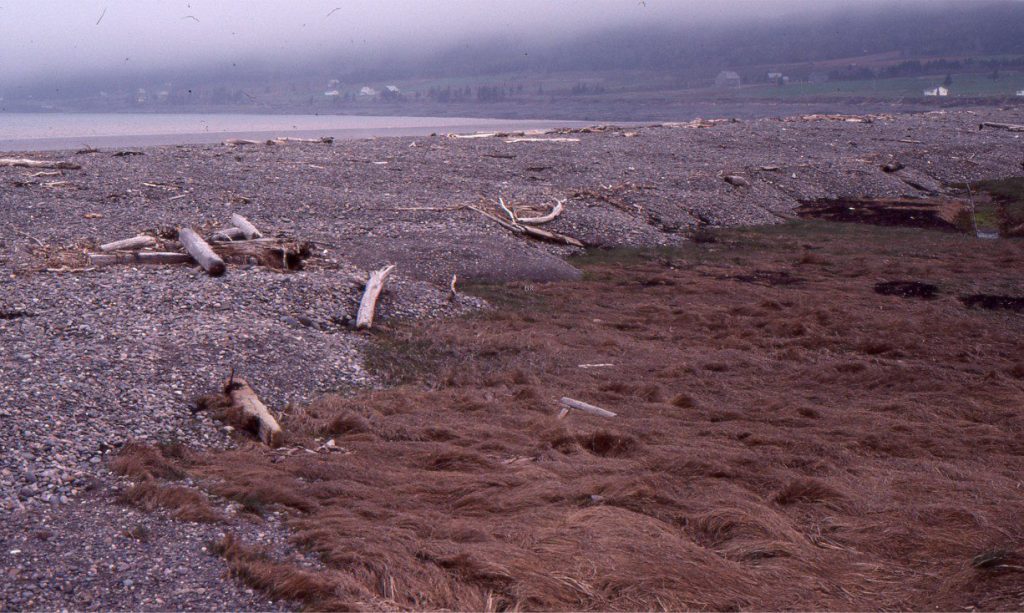
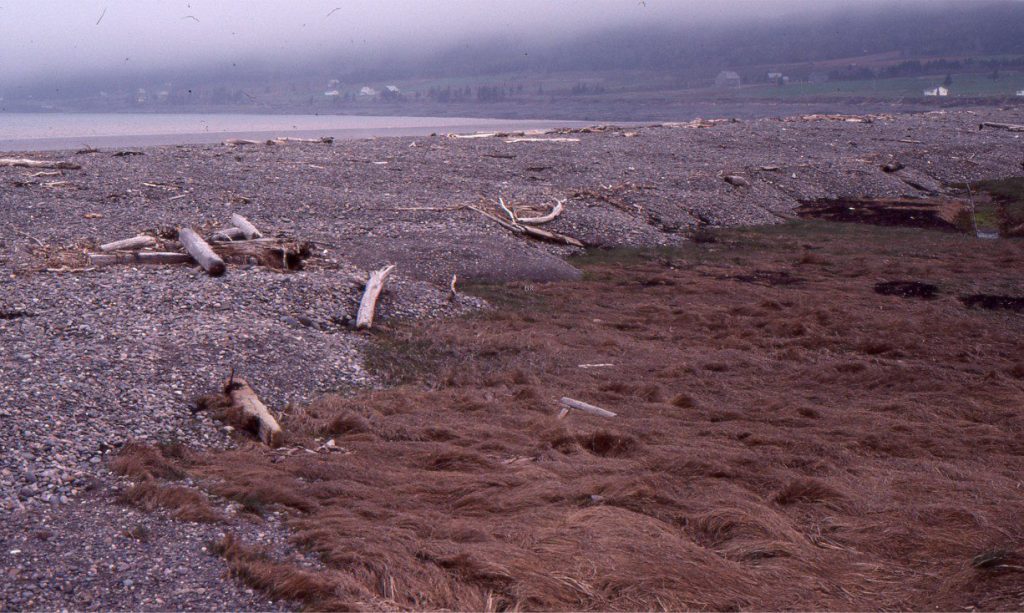
A swath of beach gravels driven by storms over the adjacent salt marsh. Cobequid Bay, an inlet in Fundy Bay, Nova Scotia. Landward migration of the beach can potentially remove all or some of the underlying salt marsh deposits.
The history of modern salt marshes begins with the post-glacial, eustatic rise in sea level that continued through the Holocene. The global trend in sea level rise will be offset by local or basin-scale differences in subsidence/uplift (particularly the isostatic response to melting ice sheets), local steric effects, sediment supply, tidal amplifications, and storminess. For example, marsh aggradation rates as high as 25.9 cm/century for the past 1400 years have been determined for some Fundy Bay salt marshes, where tidal amplification has been a major forcing factor (Shaw and Ceman, 1999).
Lithofacies associations
Two lithofacies are described: salt marsh and tidal creek. Tidal flats, mangrove wetlands, beaches, and lagoons are important as lateral and stratigraphic associations, but most of these have been dealt with in other posts.
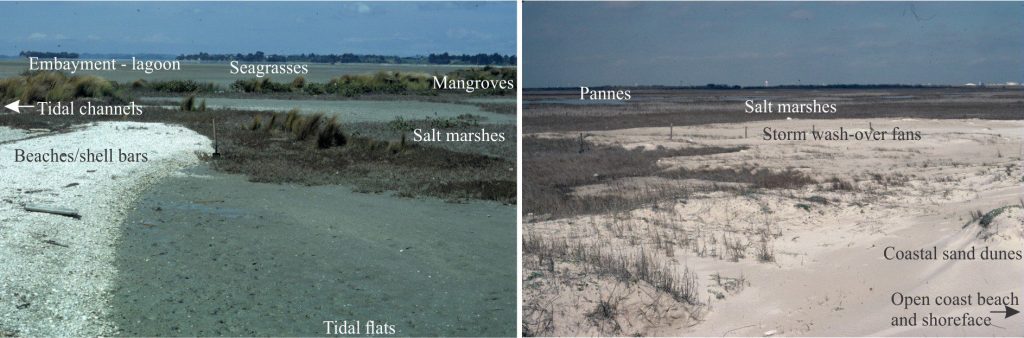
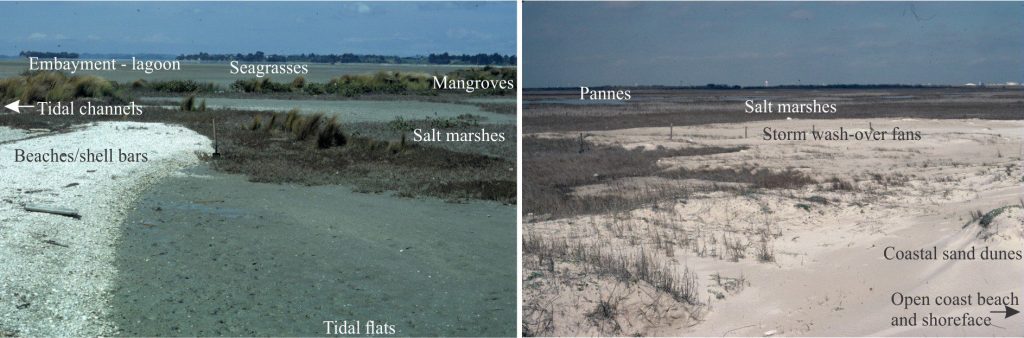
Salt marshes and their deposits rarely occur in isolation – they are usually associated with other paralic depositional settings. Two examples of these facies associations are shown here: Left: Whitford, south Auckland, and Right: Freeport, Texas. Laterally associated lithofacies have the potential to be represented in stratigraphic successions.
Salt marsh lithofacies
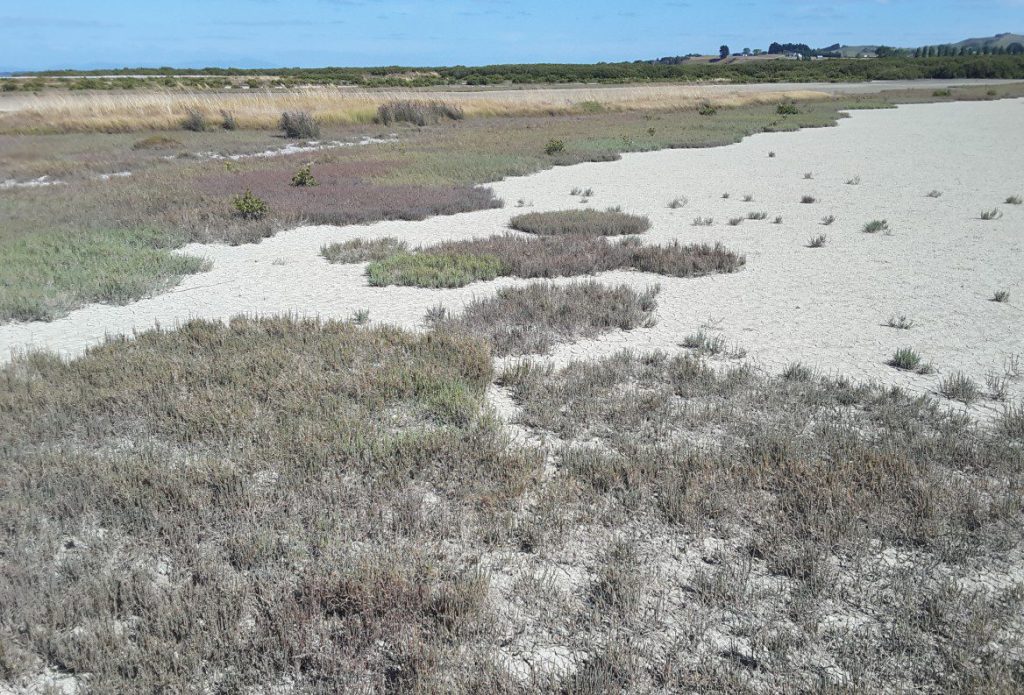
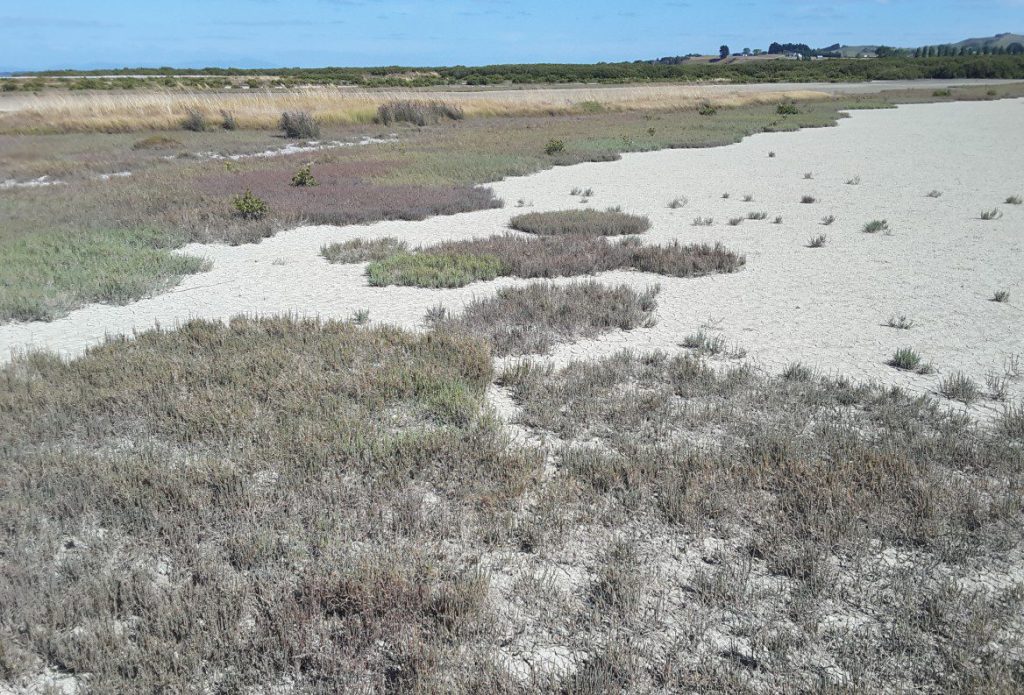
In the foreground, Salicornia-dominated, temperate climate salt marsh and intervening supratidal, mud-sand flats. In the background, pale brown sedges have taken over the marsh at slightly higher elevations. Kaiua, Hauraki Gulf, N.Z.
Salt marsh deposits can be thought of as soil profiles – they accumulate mineral sediment and organic matter, they support vegetation, an invertebrate biota and microbiota (e.g., bacteria, fungi), and are subjected to the vagaries of periodic wetting and drying. They consist predominantly of mud and silt, siliciclastic and carbonate, deposited under low energy conditions where intermittent flooding and wave activity are attenuated by the vegetation. The organic content may be concentrated in specific ‘top soil’ layers or distributed through the entire profile depending on sediment supply rates and the duration of the hydroperiod. Like fully terrestrial soils, the plant material is degraded to greater or lesser extents by anaerobic and aerobic bacterial processes, as well as grazing and foraging by invertebrate critters. Wood fragments and the roots of coastal shrubs may also be present, including the roots of mangroves in tropical and subtropical settings.
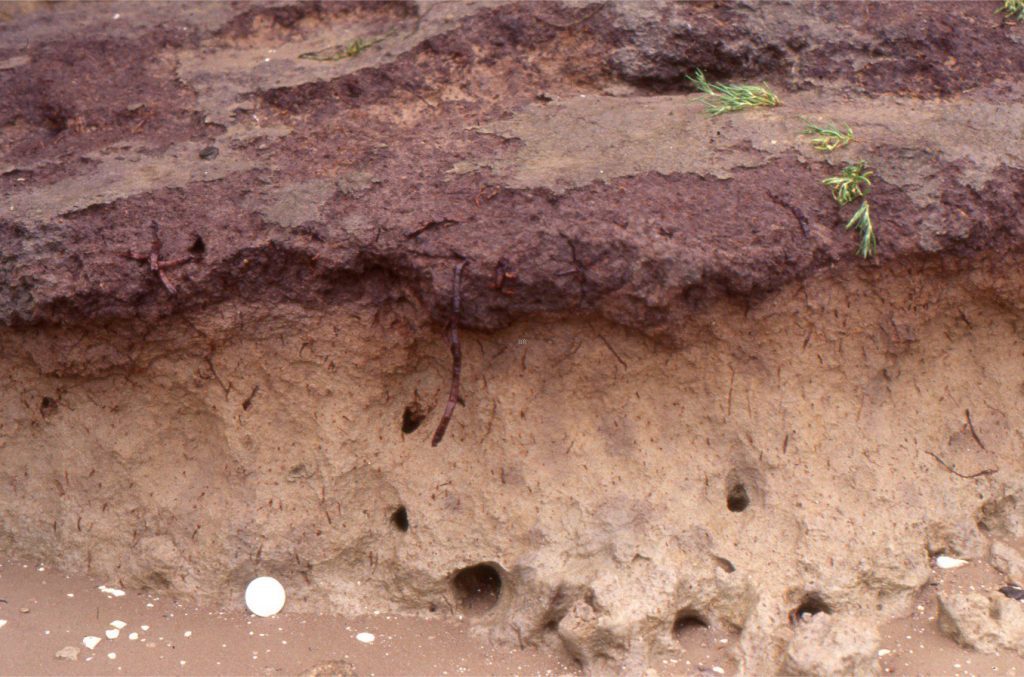
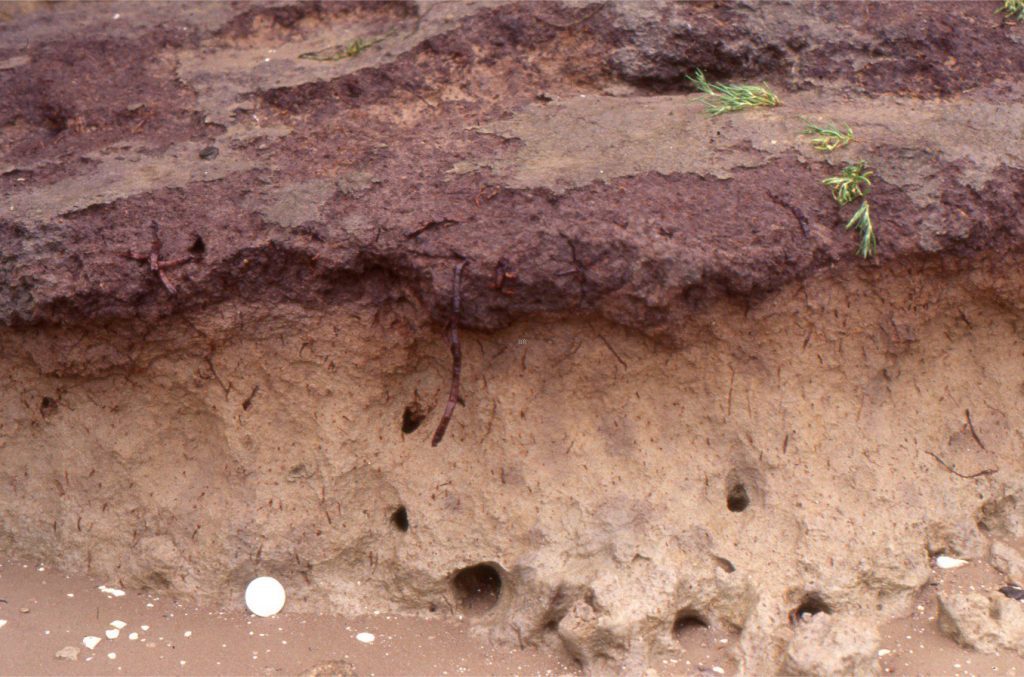
A 30 cm deep section of salt marsh consisting of silty clay (pale brown) and capping carbonaceous ‘top soil’; the contact between the two layers is gradational. There is no obvious stratification. Root structures from Salicornia and sedges abound. The large holes contain remnants of mangrove roots. Coin (lower left) is 32 mm diameter). Whitford, south Auckland.
A consequence of these depositional conditions is that traction current bedforms are usually absent. Many salt marsh profiles contain little evidence for stratification within depositional units. In others, lamination can develop – good examples from Fundy Bay, identified using X-ray radiography, are illustrated by Dashtgard and Gingras (2005) who interpret the laminae as the products of seasonal variations in sediment supply. The laminae are commonly disrupted by roots and burrows. Laminated muds may develop preferentially in pannes. Pannes that dry out will develop mud cracks and desiccated microbial mats.
Salt marsh profiles may contain multiple episodes of marsh termination resulting from storm flooding or rising in sea levels. Each episode may be bound by:
- A low-relief truncation surface (in the case of storm wave encroachment).
- Truncation of roots and burrows.
- Scattered pebbles or shell lags and lenses derived from laterally associated tidal flats and beaches.
- An organic-rich carbonaceous layer where vegetation is re-established.
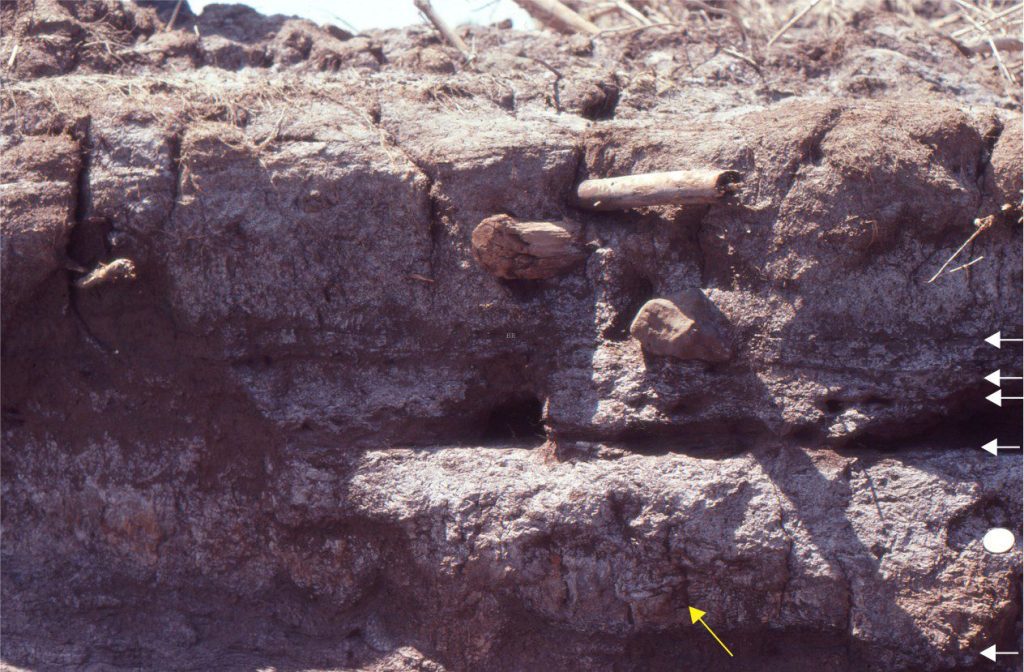
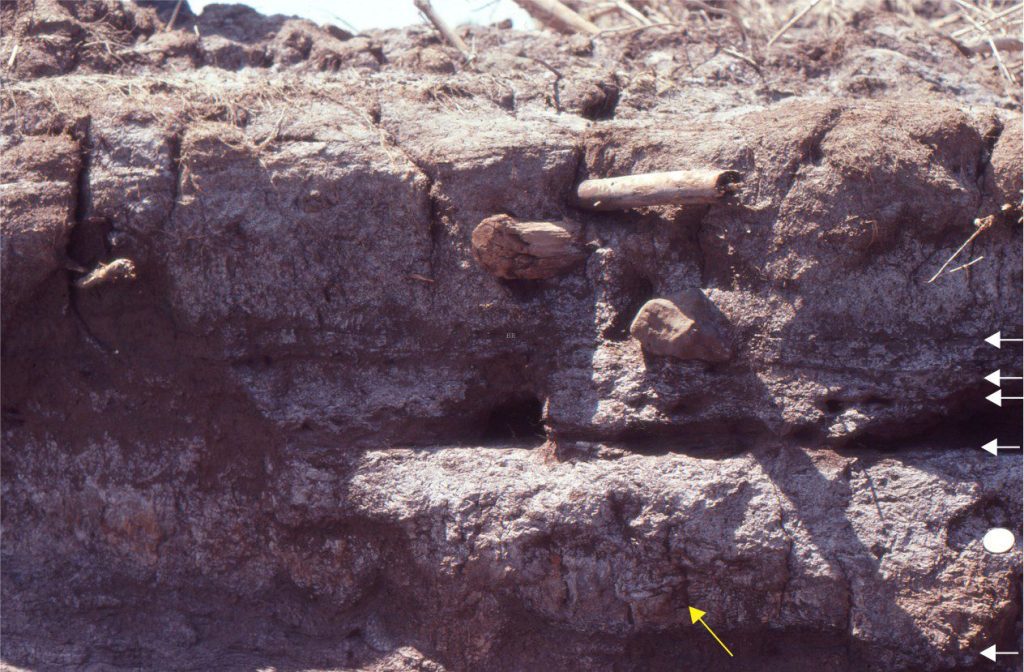
A 45 cm thick, salt-marsh cliff section reveals stacked marsh-aggradation episodes. Each episode is bound by a thin, dark brown carbonaceous band (white arrows); for each episode the overlying marsh deposits consist of clay and silt. Each episode contains root structures that penetrate the deposits of earlier deposits (e.g., yellow arrow). Larger wood fragments were probably derived from nearby coastal shrubs and trees. Coin (lower right) is 24 mm diameter. Bay of Fundy, Nova Scotia (same locality as shown in the image at top of the page).
Tidal creek lithofacies
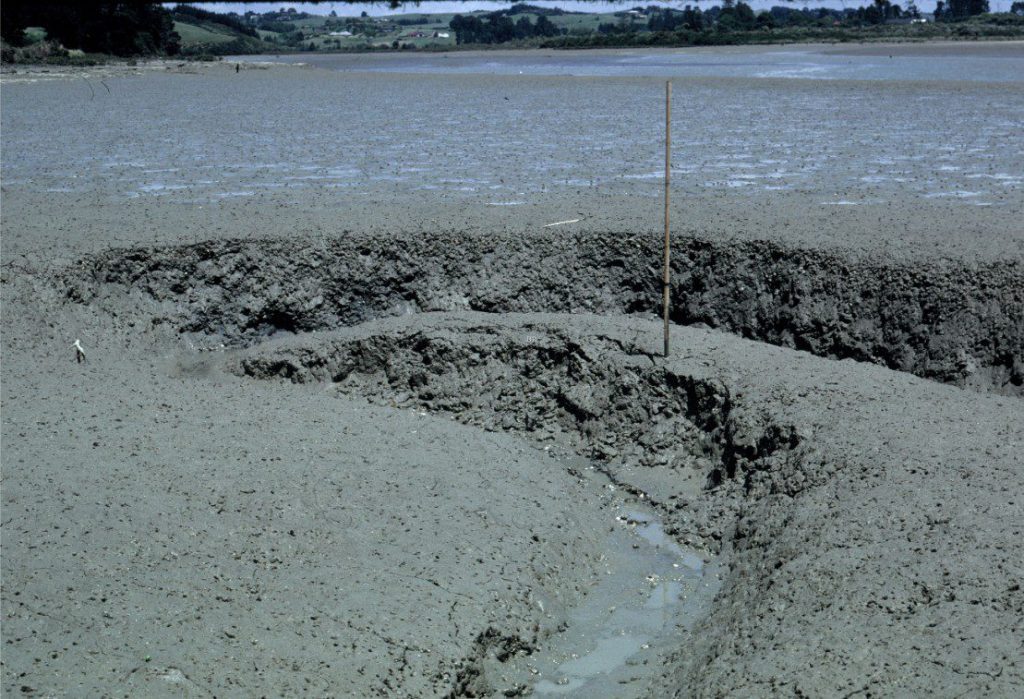
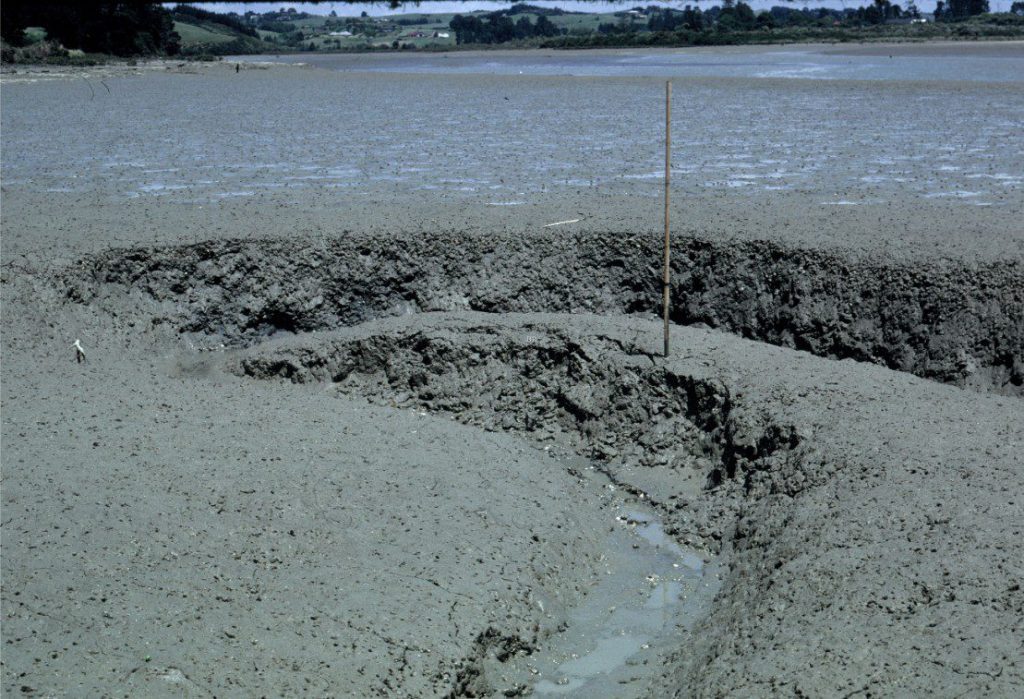
A small tidal creek just beyond the downstream limit of salt marsh, Whitford, south Auckland (about 60 cm deep). Ebb tidal flow is from image bottom to top. The sediment here is soft, grey mud that becomes dark green and anaerobic a few centimetres below the surface. The surface is littered with Amphibola crenata gastropods. Burrowing by crabs is intense – also responsible for the mottle appearance of the muds in the exposed creek margin. Small rotational slumps occur on the cut-bank (image centre). Slumped material in the creek bed is gradually dispersed by successive tidal flows.
Tidal creeks that intersect and drain salt marshes receive regular tidal flow but only to bank-full levels during spring tides. Most of the creeks terminate over the marsh surface and there is no continuous through-flow like that in larger tidal inlets and estuarine channels. Consequently, creek sediment is dominated by muds and silts, with the introduction of some sand and gravel during high energy storm events.
Deposition in small creeks, like that shown above, will be dominated by massive or weakly laminated muds and pockets of chaotic blocky muds derived from rotational slumping of creek margins. Burrowing by a variety of invertebrates may completely obliterate any pre-existing sedimentary structures. Roots may extend into the channel margins.
Larger channels show a more diverse array of sedimentary structures including:
- Large-scale, shallow dipping laminae consisting primarily of silty muds and thin sandy beds; the dipping beds are analogous to point-bar foresets.
- Ripples and small pebble-filled scours.
- Thin organic-rich layers, possibly with root structures.
- Dipping beds merge with slump packages that form on the steeper cut banks.


A schematic tidal creek profile oriented normal to the channel axis, based partly on the Whitford creek image above, and partly on inference. The dominant lithofacies is clay and silty clay, with some thin sandy veneers along point-bar foresets. The rotational slump glide-planes terminate at the creek floor.
Regular tidal flooding in the creeks means that they can support a more diverse invertebrate fauna – not only burrowing crabs, worms, and shrimp, but also bivalves and gastropods. For example, Holocene creek deposits in Fundy Bay contain passively filled Mya arenaria and Macoma balthica bivalve burrows several centimetres deep. Worms and shrimp can construct more complex U-shaped burrows with meniscate fills and linings (Dashtgard and Gingras op cit.).
Stratigraphic trends
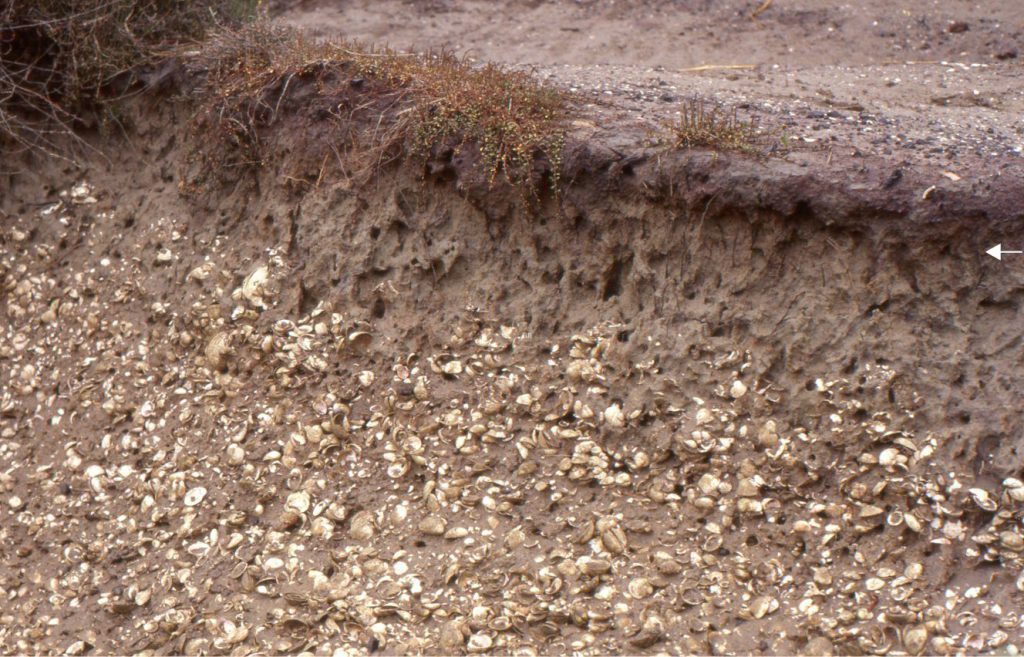
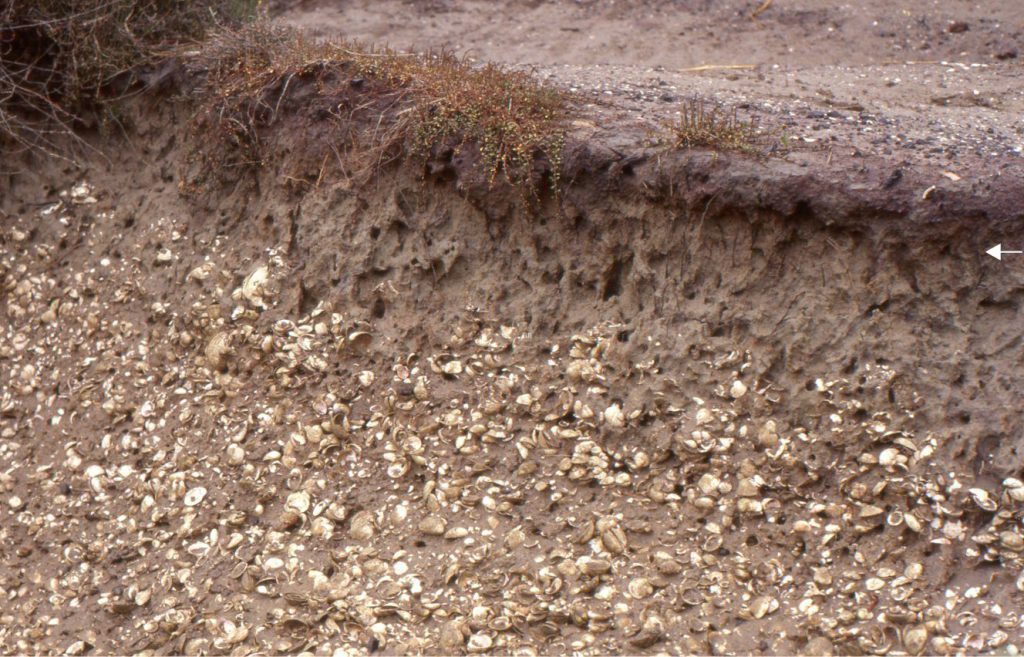
A stratigraphic section through shell-sand tidal flat and overlying salt marsh clay-silt deposits, capped by a plant-rich organic layer. Root structures in the marsh deposits extend into the top of the shell-sand. The marsh also contains simple vertical burrows, probably excavated by crabs. The tidal flat molluscan fauna is dominated by venerid bivalves. The deposit is late Holocene in age. The section is exposed in a dug canal. Whitford, south Auckland.
Salt marshes, like other coastal paralic depositional settings, occur at the intersection between marine shelf or platform and terrestrial environments. Their preservation and stratigraphic representation depend on whether the depositional system is regressive and prograding, or transgressive and retrogradational. A typical stratigraphic profile for normal regression and progradation is shown below.
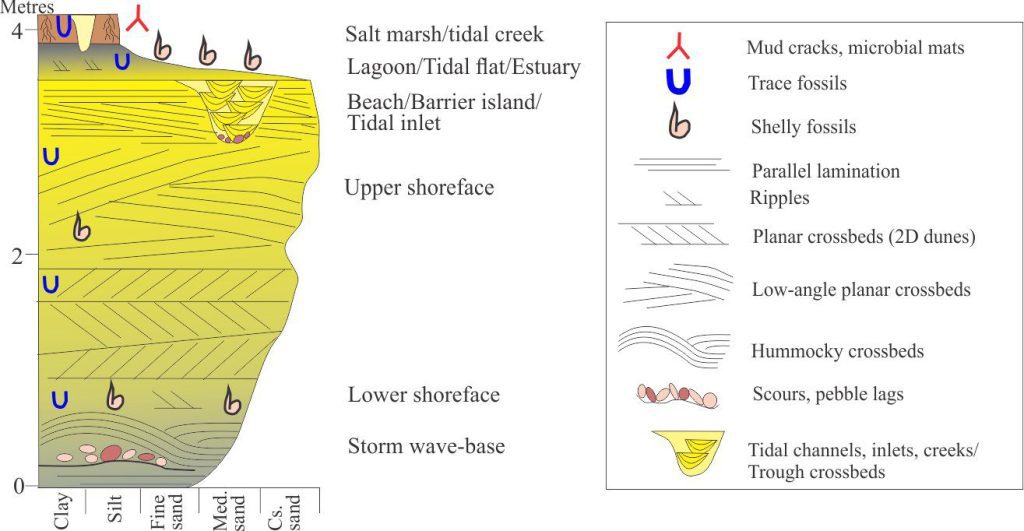
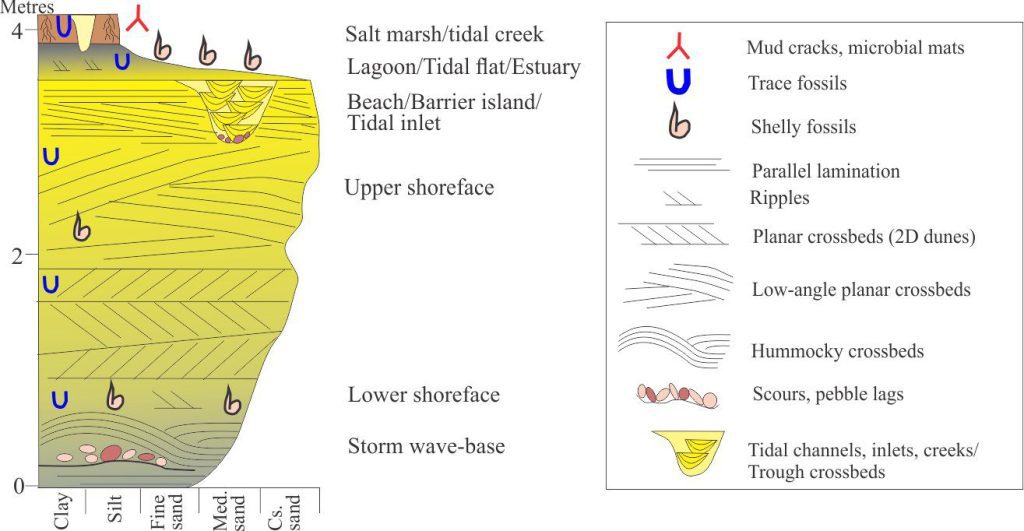
A typical coarsening-upward lithofacies motif that can develop during normal regression and coastal progradation (deeper shelf not included). The upper part of the column represents common paralic depositional environments at the transition from marine to non-marine conditions, culminating with salt marsh deposits. Shoreface ravinement during transgression may remove all or some of the marsh deposits.
Stratigraphic trends that indicate progradation are commonly represented by coarsening upward or bed-thickening upward successions that record the progression from relatively deep water (for example below storm wave-base) to shoreface, and culminating with the deposits of paralic environments that in this case includes salt marsh. The stratigraphic trend is exemplified by an upward- progression in grain size and bedforms (particularly ripples, subaqueous dunes, HCS) that reflect increasing wave and current energy across the sea floor, and changes in biota – invertebrates and their trace fossil record. Ideally, the upper part of the succession might include evidence for subaerial exposure (e.g. scour surfaces, desiccation structures, beach rock).
[paralic here refers to any depositional system at the junction of marine- terrestrial environments: beach, barrier island, coastal dunes, lagoon, estuary, tidal flat, coastal plain, delta interdistributary bay].
Shoreline retreat during a rise in relative sea level will drive the landward excursion of salt marshes – the survival of the marsh system will depend on whether they can keep up, catch up, or give up as a consequence of inundation. Marsh cliffs will retreat under constant attack by waves. The preservation potential of salt marshes during transgression (like most other paralic depositional systems) is also reduced by erosion beneath the shoreface as it migrates in tandem with the shoreline. Shoreface ravinement can remove all or some of these deposits.
Here’s the link to posts on other lithofacies

















