









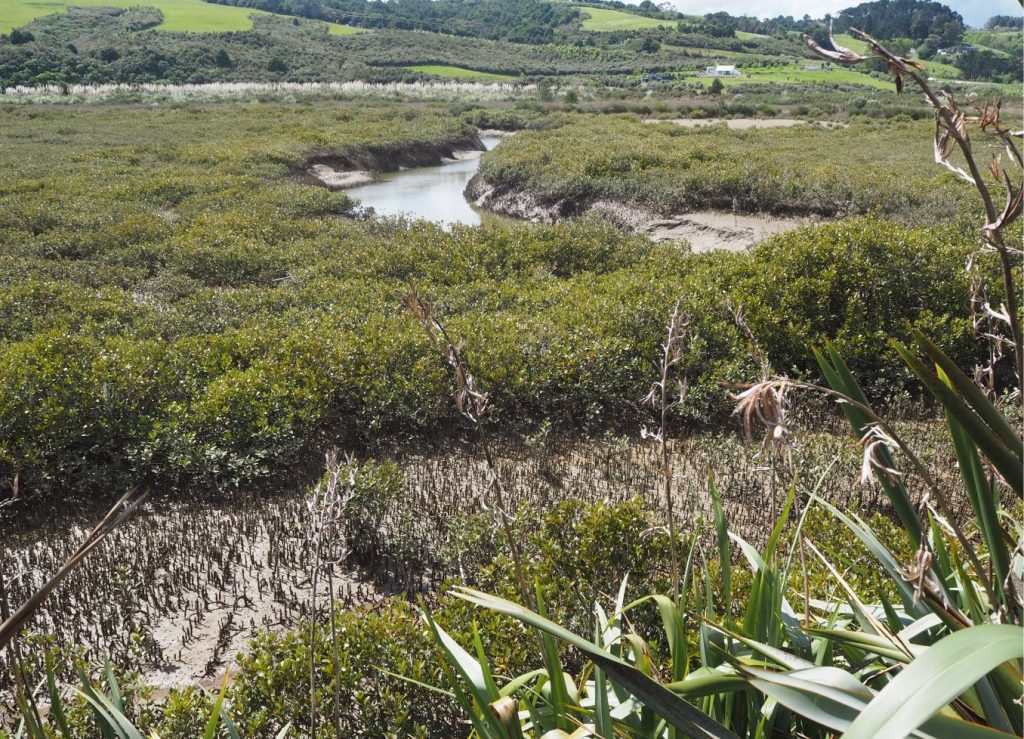
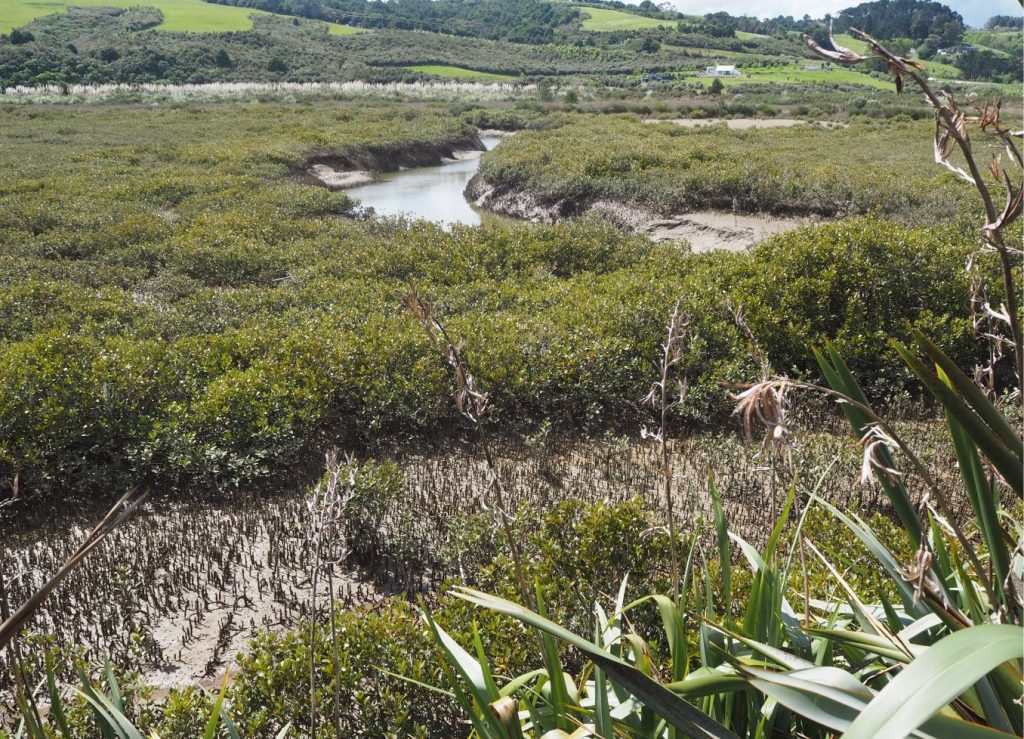
Mangroves dominate this estuarine channel-tidal flat system. The channel is mud-bound, about 1.5 m to 2 m deep and 5 m to 6 m wide, with margins stabilized by the tangle of roots. Tidal flood waters cover the vegetated flats to depths of only a few centimetres. Each tidal cycle replenishes the supply of food and nutrients to the vegetation and the local invertebrate fauna. Whitford estuary, south Auckland.
This post is part of the Lithofacies Series.
Mangrove trees and forests occupy a very specific zone between fully marine and terrestrial environments in supratidal and upper intertidal realms. They are tolerant of both saline and freshwater conditions but tend to favour the former because there is less competition from terrestrial plants. Their trunks and roots tolerate periodic immersion in seawater (tides), but not permanent subtidal submergence. They prefer low wave- and tide-energy, and muddy substrates; established forests exacerbate the muddiness because they damp waves and tidal flows, and because they add significant quantities of organic detritus to the sediment.
Mangroves are restricted to ocean surface temperatures greater than 15o C such that their latitudinal extent is about 30o N and 38o S. Their tropical to warm-temperate range is reflected in species diversity (greater in warmer environments), and the diversity of associated floral (mostly algal), invertebrate, and vertebrate contributions to mangrove ecosystems. At their southern extent in New Zealand mangroves are represented by a single species – Avicennia marina.
Mangrove evolution
Mangroves are angiosperms. The evolution of angiosperms in the Late Cretaceous is commonly referred to as the Cretaceous Terrestrial Revolution (Benton et al., 2021 OA). This event changed everything – insect evolutionary trends, plant dispersal, a restructuring of the food web and consequent evolution and dispersal of vertebrates and invertebrates, and patterns of sedimentation in environments associated with vegetation. Mangroves, with their specialized adaptations, probably appeared a bit later in the Cretaceous, and may have reached some kind of niche development threshold during the Paleocene-Eocene thermal maximum (PETM), with the appearance of modern genera like Avicennia and Rhizophora. One hypothesis proposes that mangroves developed their tolerance for saline conditions during the PETM when glacio-eustatic sea levels were high and coastal lowlands were inundated. There is evidence that Avicennia grew in coastal wetlands as far north as 75o N in Siberia and Arctic Canada during this period (Salpin et al., 2019, PDF available). Seed dispersal by ocean currents was probably an important part of mangrove dispersal, but so too were the longer-term plate tectonic changes to continent and ocean configurations with the breakup of Gondwana continuing into the Late Cretaceous and Paleogene, the opening of North Atlantic Ocean and closure of Tethys.
Lithofacies character
Attenuation of waves and tidal currents by mangrove trunks, roots, and pneumatophores results in low-energy depositional conditions dominated by mud and silt, and generally lacking in structures formed by bedload transport of sediment. Any depositional layering will likely be disrupted by growth of roots, pneumatophores, and burrowing by worms, molluscs, and crustaceans (particularly crabs and shrimp). These basic sedimentological characteristics apply to terrigenous- and carbonate-dominated sediments. The organic content of the muds is variable and in outcrop is represented by lithologies ranging from carbonaceous mudstone to coal.
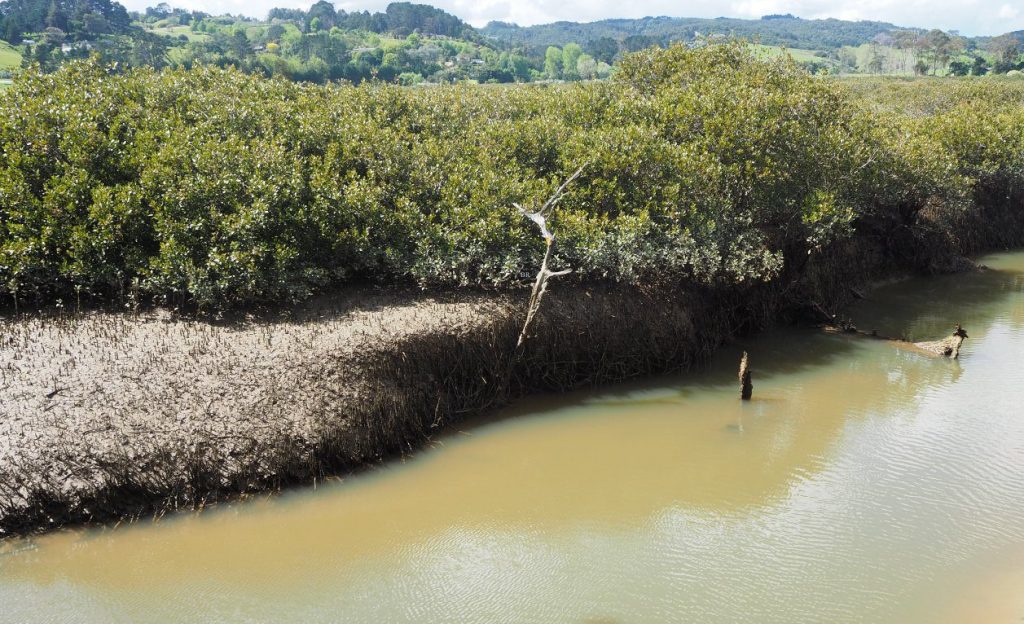
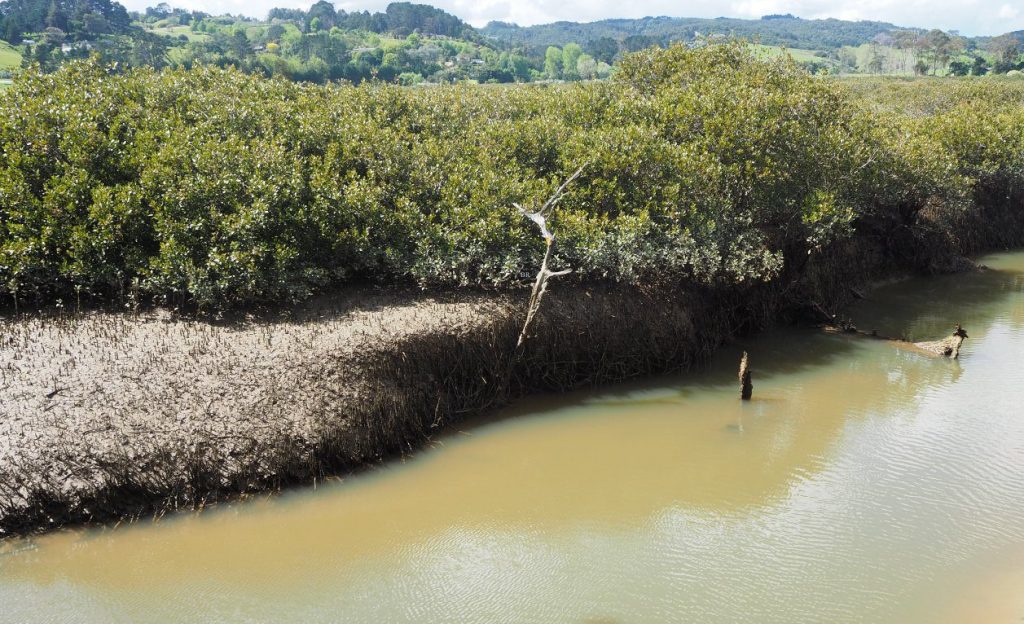
Mangrove wetland substrate is exposed in this tidal channel margin. Except for a few millimetres of grey-brown oxidized mud at the surface, the entire section, about 80 cm thick, is composed of dark blue-grey mud bound by a dense tangle of tree roots and pneumatophores. The root mass helps stabilize the channel bank. The exposed surface is littered with grazing gastropods (Amphibola crenata). Whitford estuary, south Auckland.
Fossil content
A diverse assemblage of benthic invertebrates inhabits the sediment-water interface, burrows beneath its surface, or are attached to exposed roots and pneumatophores. Species diversity is greatest in tropical settings, however, only those with hard shells have the potential for preservation. Crustaceans such as crabs and shrimp and annelids (worms) may leave preservable surface traces or burrows. Crab burrows for example are mostly simple, vertical, passively filled tubes. Shrimp burrows may be Skolithos-like. In New Zealand, nereid worms that are common in coastal wetland muds create U-shaped burrows (Morton and Miller, 1973).
Oysters and mussels that encrust or attach to roots and pneumatophores will probably become detached when either the animal or the woody substrate dies and disintegrates. This may also apply to encrusting foraminifera. Encrusting species like oysters may preserve bioimurred impressions of the wood.
Unfortunately, many of the invertebrates and protozoa that inhabit mangroves also thrive in other intertidal environments. However, mangrove pollen, if preserved, provide unequivocal evidence, particularly when found in association with the other sedimentological features. Mangrove pollen have much greater preservation potential compared with mangrove leaves and wood. Pollen abundance is potentially high in carbonaceous or coaly lithologies. Hisham and Abidin (2023) found this to be the case in Malaysian Early-Middle Miocene coastal wetland deposits, where Avicennia, Rhizophora, and Florschuetzia pollen are abundant.
Despite the abundance of roots in modern mangrove environments, their representation in the rock record is poor. Woody material deposited on the surface is prone to disintegration; in the subsurface it is prone to degradation by anaerobic bacteria. Physical compaction and dewatering during burial will also result in fundamental changes to roots structures. For example, a metre-thick mud when buried 2-3 km will appear in the stratigraphic record as a mudstone only a few centimetres thick. Likewise, compaction of organic rich, coaly layers will reduce original thicknesses by 10 to 20 times. Under these circumstances, the identification of primary root structure is difficult.
A cautionary tale is presented by Perry et al., (2008), who note that encrusting foraminifera, shelly invertebrates, and woody matter that are common in some modern Australian mangrove wetlands, are not preserved in older Holocene deposits – it appears that the carbonate skeletal components may have been dissolved, and that the organic remains altered by aerobic or anaerobic processes.
REDOX conditions
Dig into mangrove wetland muds and you will soon discover the fetid, greenish-black, oozy deposits that are typical of wetlands everywhere. Muds near the surface have varying amounts of oxygen to depths of a few millimetres to centimetres. Below this layer, the low-permeability muds remain permanently saturated and oxygen diffusion is negligible, conditions that promote chemical reduction. There is a progression of redox reactions once oxygen has been depleted, promoted in part by the metabolic activity of bacteria in the muds (Pezeshki and DeLaune, 2012): nitrate is reduced to nitrogen, reduction of manganese (to Mn2+) and iron (to Fe 2+), sulphate to sulphide (that can combine with Fe2+), and CO2 to methane (hence swamp gas, will-o-the-wisp).
The carbon dioxide component can be generated by anaerobic bacterial breakdown of plant matter, a process that may explain the paucity of fossil mangrove macroflora in the rock record. The lithofacies record of these redox conditions can be preserved as black or bluish- green mudstone-shale in association with carbonaceous or coaly mudrocks. Most invertebrates tend to inhabit the shallowest oxygen-prone deposits.
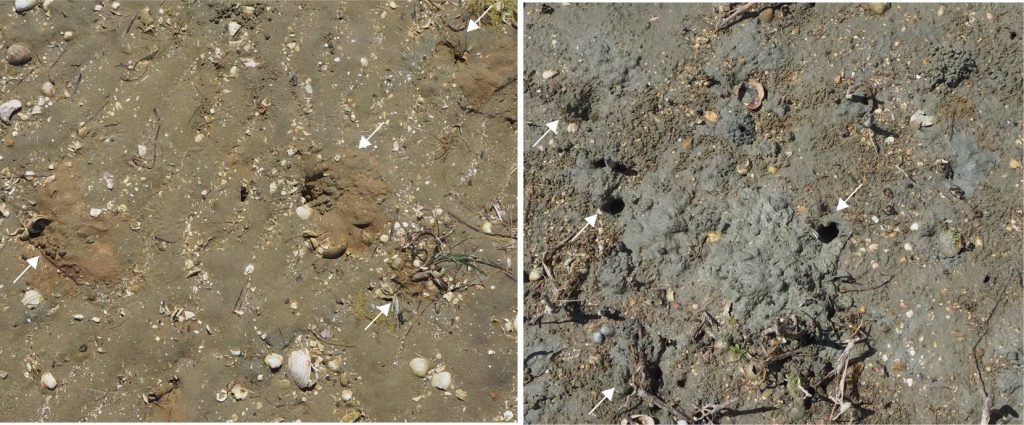
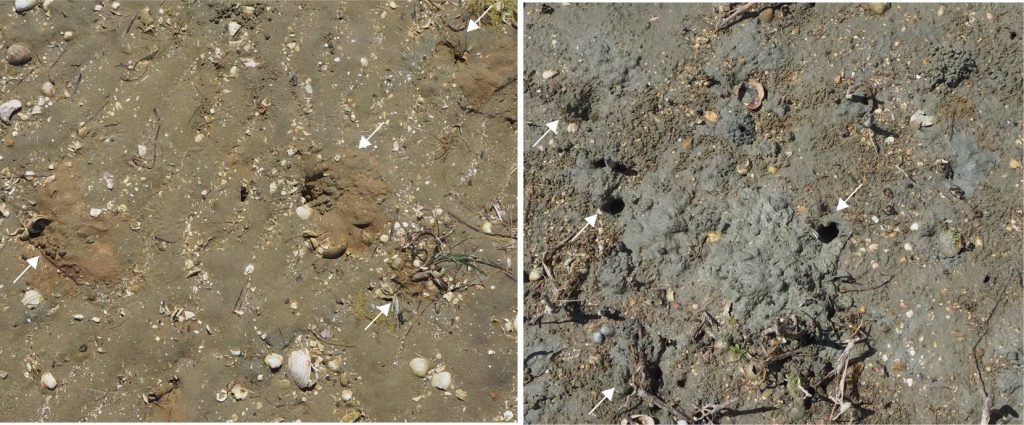
Excavation by burrowing crabs (arrows) reveals differences in the redox conditions of the substrate. On the left, the excavated sediment on a non-vegetated tidal flat is brownish-grey and much the same colour as the tidal flat sediment. The same species of crab has excavated reduced blue-grey mud in substrates associated with mangrove stands.
Lateral facies associations
Mangrove forests and coastal fringes are bound seaward by intertidal areas – tidal flats in lagoons, embayments (including delta interdistributary bays), behind detached beaches and coastal sand dunes, and the seaward continuation of estuarine channels. Sediment compositions range from mud- to sand dominated, with all manner of combinations in between depending on:
- Coastline shape – this is particularly important in seaways having highly embayed and indented coasts, as is commonly the case for drowned valley geomorphic systems and embayments protected by barrier islands and bars.
- Tidal range and tidal energy.
- The availability of sand.
- The capacity for tidal currents and waves to disperse the coarser grain-size fractions, including the capacity for creating ripple and dune bedforms.
- The damping effects of seagrass meadows, if present.
Sediment mineralogy can be carbonate- or siliciclastic-dominated, or mixtures of these two. Carbonate intertidal environments commonly contain abundant invertebrate skeletal and calcareous algal fragments, ooids, carbonate mud, microbialites, and detrital gypsum-halite in hypersaline environments. There is a range of sediment bedforms, trace fossils, marine invertebrates, and calcium carbonate secreting algae that provide excellent tools for identification and interpretation of these non-vegetated intertidal lithofacies.
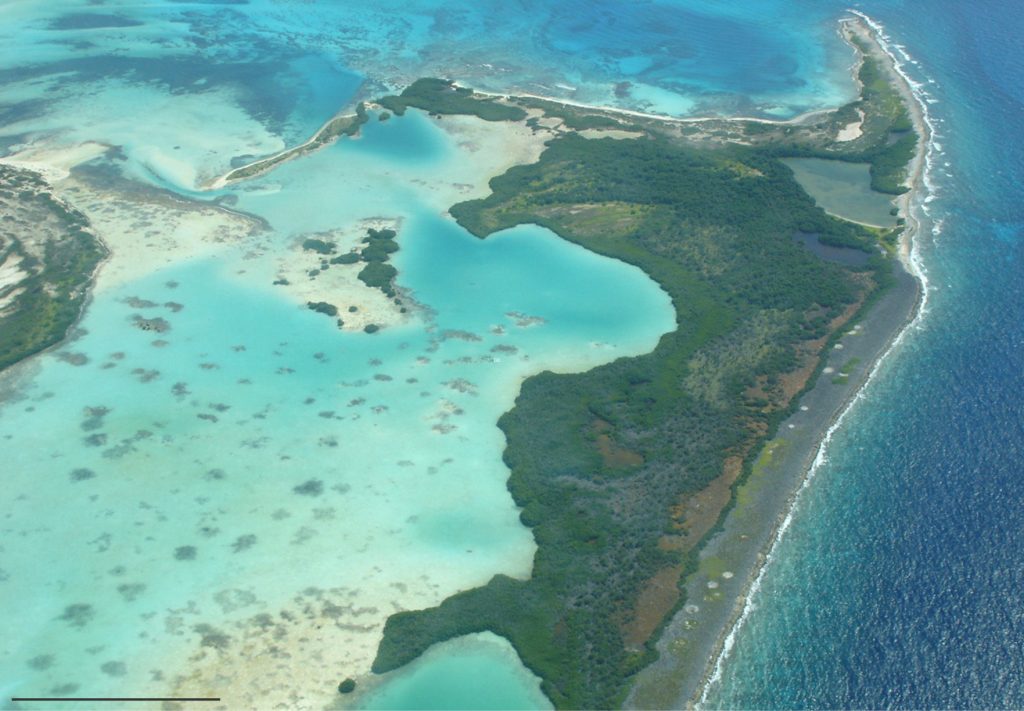
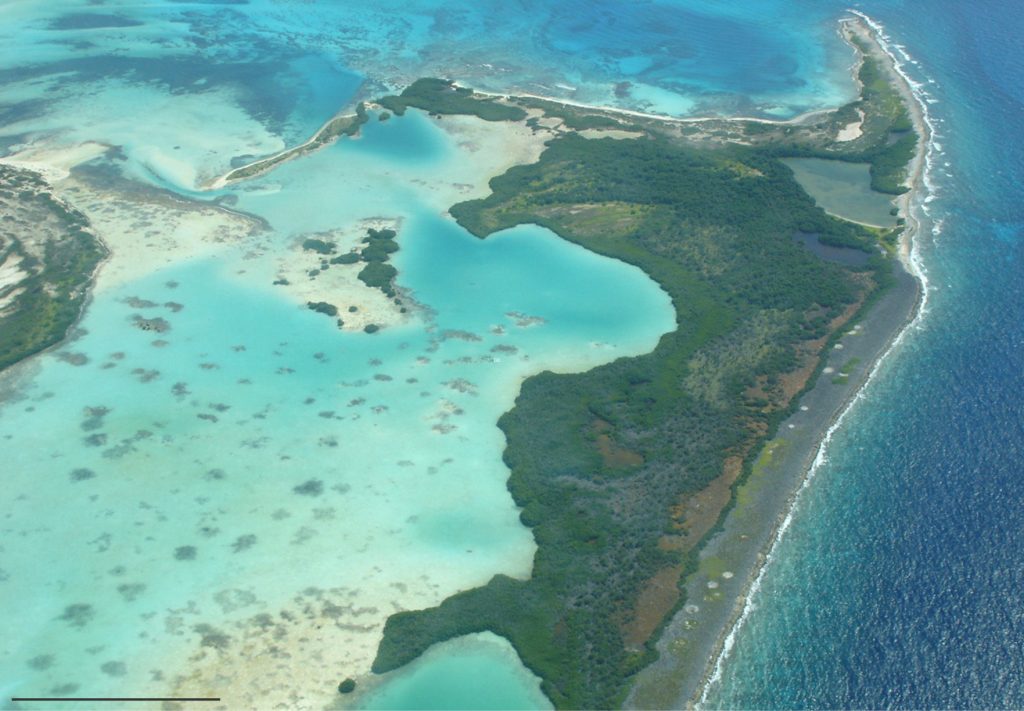
Part of the southern barrier reef on Los Roques Archipelago, Venezuela, emphasizing the back-reef that contains mangroves (Rhizophora mangle) and salt marshes that fringe lagoons with seawater salinities ranging from normal to hypersaline. Sediment beneath the mangroves is anaerobic, and exposed tidal flats commonly develop microbial mats and thrombolites. Distance scale at bottom left is 250 m. Image and information courtesy of José Alejandro Méndez.
In the examples below, the tidal flats are mixed siliciclastic sand-mud with abundant wave and current ripples, and low amplitude bars consisting of disarticulated and fragmented bivalves and gastropods. Seagrass meadows may also be present in intertidal to shallow subtidal environments.
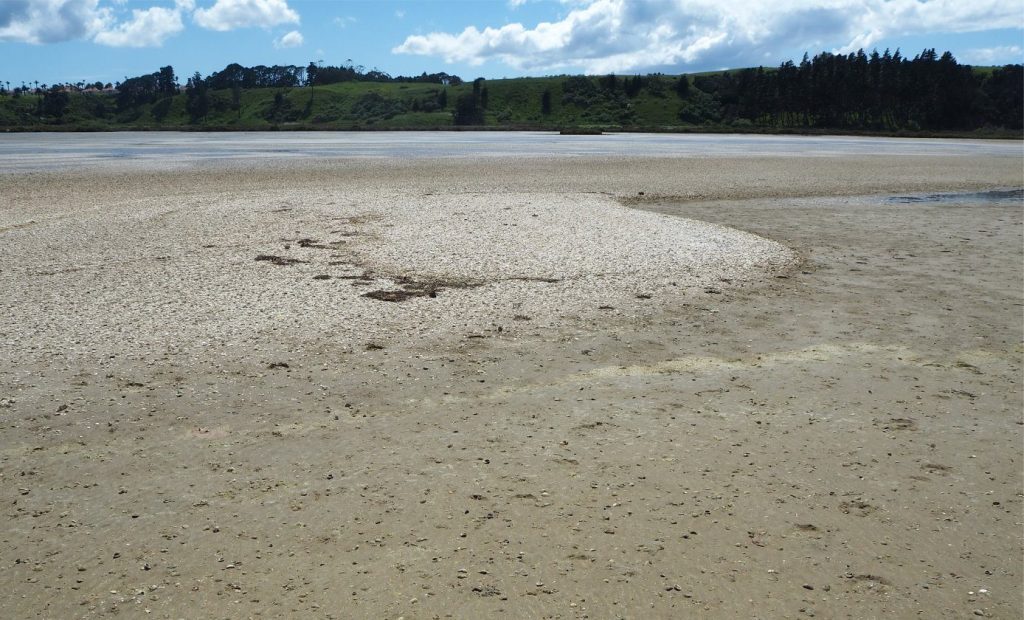
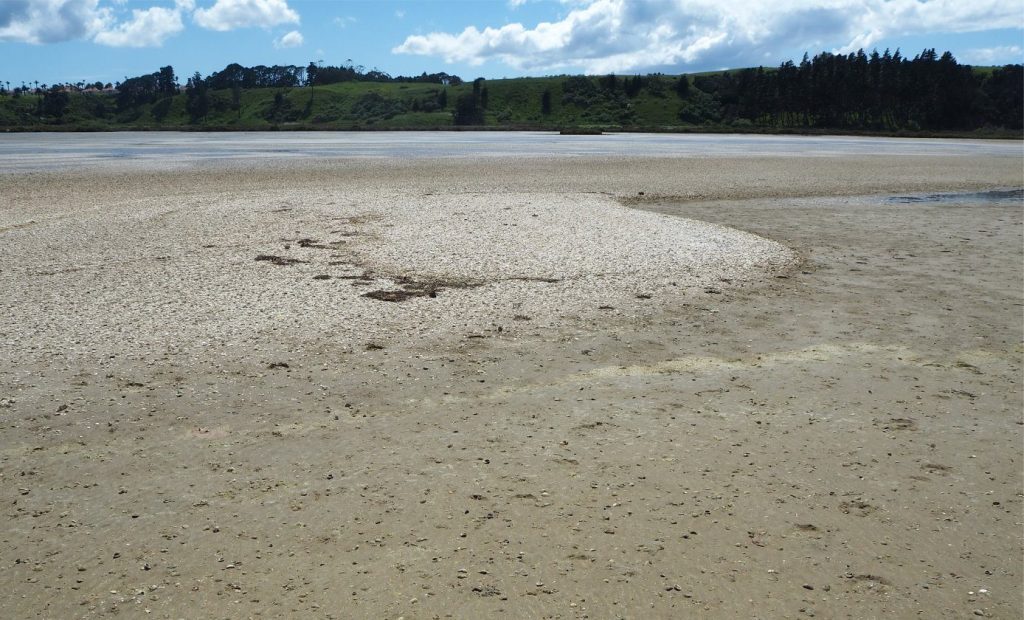
Broad tidal flats develop on the seaward margins of mangrove forests, and in this example, large bedforms include shell banks and bars. The shells are dominated by disarticulated and broken venerid bivalves and subordinate gastropods, all of which have living relatives that inhabit the tidal flats. Other sedimentary structures include a variety of wave and current ripples, and an abundance of crustacean, worm, and mollusc trails and burrows.
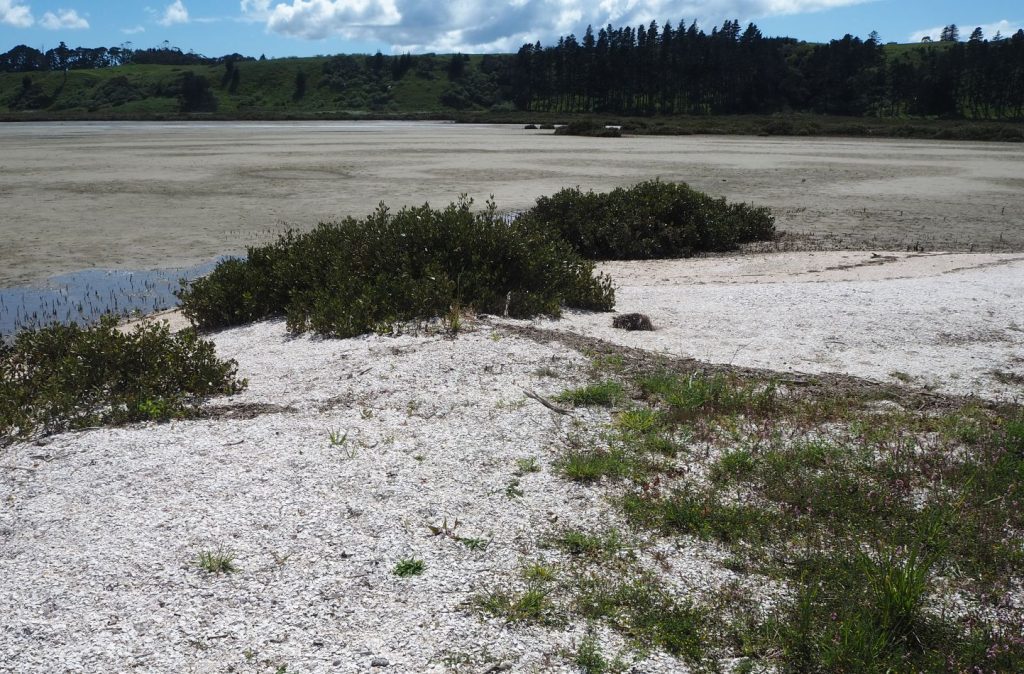
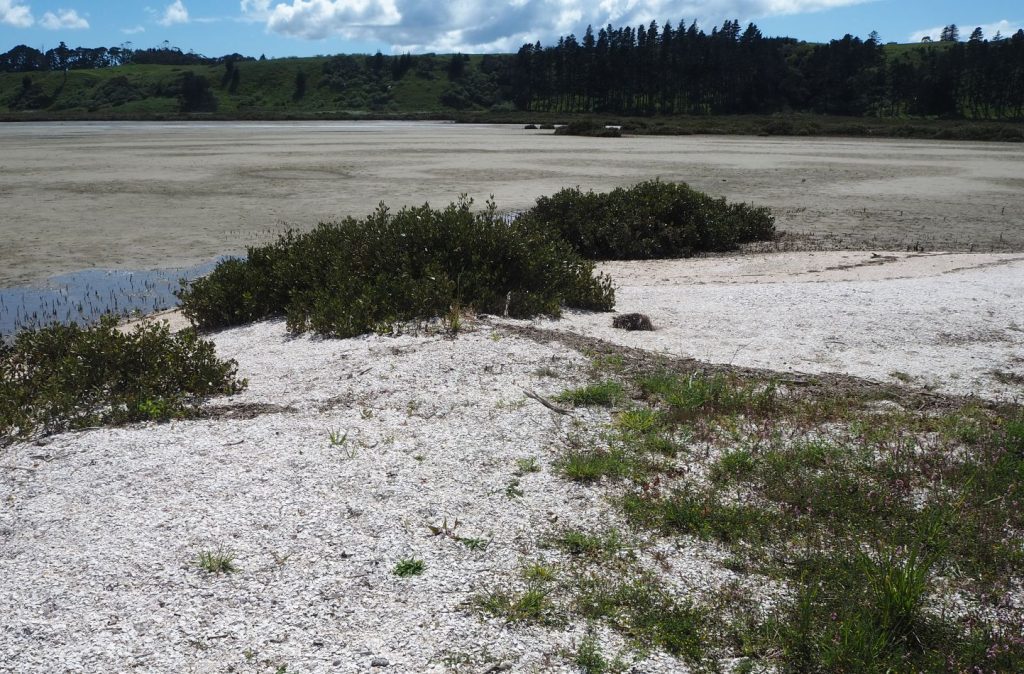
The transition from mangrove wetland to fully marine tidal flat lithofacies in this example is distinguished by shell bars, and shell-strewn beaches that are marginal to salt marsh fringes. The abundance of sandy deposits, shelly invertebrates, crustaceans, and bedforms that indicate tidal reversals is in marked contrast to the sedimentological content of mangrove substrates.
Mangrove stands may pass landward directly to terrestrial flora and/or fluvial environments, or to salt marshes that tend to accumulate in upper intertidal to supratidal settings bound by spring tide limits; they are not inundated except at the highest tides. Salt marshes are widely distributed globally, as far north as Greenland and Iceland and south to the Sub-Antarctic Auckland and Campbell islands. Their vegetation is characterised by salt-tolerant succulents like Salicornia and grasses like Spartina (cord grass). Many species of gastropod that graze or scavenge amongst mangroves and tidal flats do not venture into the salt marshes. However, burrowing crabs and worms seem to like this environment and they can be the dominant marine invertebrate there. Salt marsh soils are better drained than their mangrove counterparts with higher concentrations of oxygen. This may be preserved in the rock record as a lateral transition from significantly reduced sediment to more oxidized sediment; stratigraphic profiles will potentially reflect this redox transition.
Salt marsh soils also contain a meshwork of roots of succulents, grasses, nearby mangroves, and other coastal shrubs. Roots have relatively low preservation potential although they may be preserved as carbonaceous outlines or sediment infills. The combination of root growth and invertebrate burrowing means that any primary layering will be disrupted.
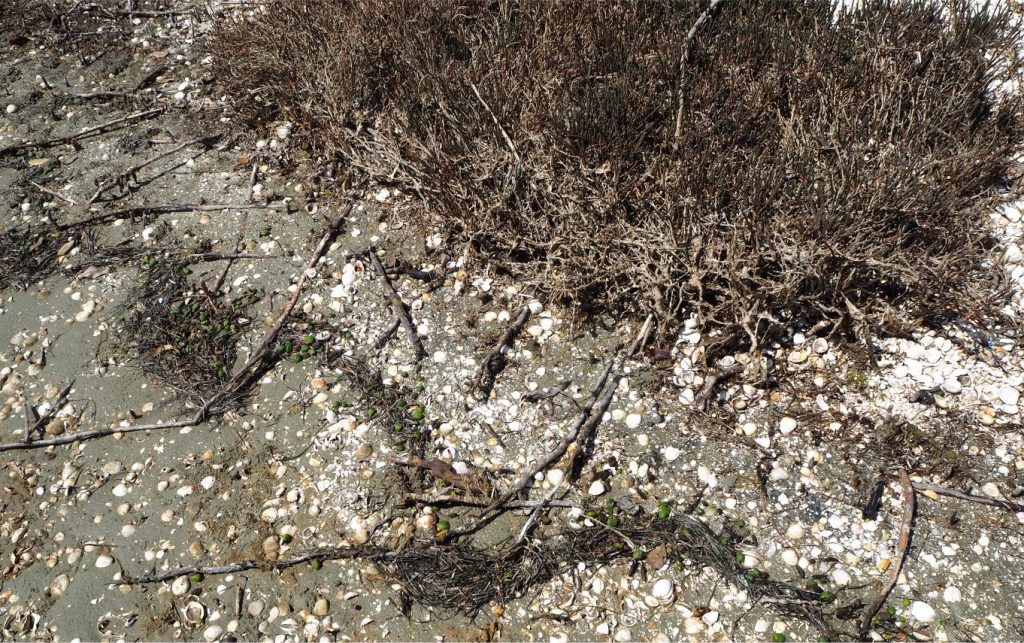
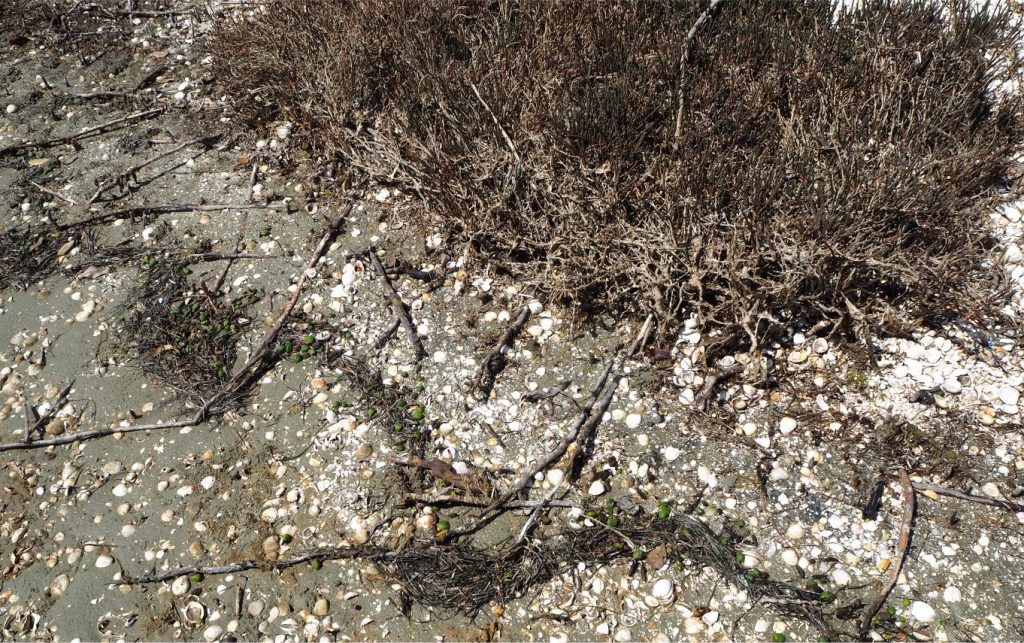
A tangle of salt marsh Salicornia roots (fine root structure) interwoven with larger, longer mangrove roots within the salt marsh deposits. In this example there is a significant admix of disarticulated and broken shells near the facies transition with the upper tidal flat. Whitford, south Auckland.
Stratigraphic profiles
A consequence of shoreline excursions during changes in relative sea level is that laterally associated lithofacies will be represented stratigraphically in profiles that record shallowing or deepening upward conditions. This is the essence of Walther’s Law. For example, an ideal shallowing upward, shelf to shoreline profile might show:
- At the base of the profile, the transition from mud-dominated outer shelf to sandy inner shelf with bedform indications of wave-base processes, to
- Upper shoreface and intertidal depositional conditions.
- Regardless of the paleoenvironmental setting, mangrove lithofacies should occur either at the top of the marine stratigraphic succession, or at the transition from marine to non-marine.
How mangroves respond to sea level changes will depend on factors such as
- The rate of sea level change. Rapid sea level rise may result in drowning of mangrove stands.
- As rates of sea level rise decline, mangroves are more likely to play catch-up of keep-up with the landward-migrating intertidal zone (e.g., Woodroofe, 2018).
When relative sea level falls, the intertidal zone will migrate seaward. The rate at which mangroves will colonize this zone will depend on sediment supply as well as the rate of sea-level change. A good example of rapid shoreward accretion of tidal flats and mangrove development has been documented along the Firth of Thames at the south end of Hauraki Gulf (New Zealand). The coast there in 1950 contained a broad, sandy tidal flat, that by 2007 had been covered by a 1000 m wide band of Avicennia marina; the non-vegetated tidal flat had prograded seaward in response to this floral takeover (Lovelock et al., 2010, PDF available). Shoreline accretion here is largely the result of high sediment flux to the Firth, a consequence of surface runoff from land use changes and deforestation.
Links to the companion posts
Seagrass meadows and ecosystems
Seagrass lithofacies in the rock record

















