









Glauconite – an important stratigraphic and paleoenvironmental indicator
Glauconite is one of the most recognizable minerals in the entire sediment suite, primarily because of its green hues, its common peloidal grain morphology, and microcrystalline structure. Green-ness does apply to a few detrital minerals like amphiboles, pyroxenes and chlorite, but they can be distinguished by their crystal habit and prominent cleavage; sedimentary glauconite is always microcrystalline.
Glauconite is a sheet silicate, distinctive in its high concentrations of Fe2+, Fe3+, and K. It occurs as different types depending on the relative proportions of Fe and K. Well-ordered (mature) glauconite is high in K (8% to 9%, derived from sea water) and its crystal structure is closest to that of micas. Precipitates with lower K contents tend to have more clay-like structure, particularly smectite (a mixed crystallographic bag of expandable clays). Total Fe is commonly >15%, and the amount of Fe3+ is usually > Fe2+ (B. Velde, 2014). Glauconites with low K and high Fe tend to have brown-yellow hues. Most glauconites begin precipitation as K-poor smectites that, over about 105 to 106 years, become increasingly K-rich and crystallographically ordered, or mica-like (Odin and Matter, 1981). The increase in crystallographic ordering can be measured using X-ray diffraction.
Glauconite is a marine, authigenic mineral that precipitates at very shallow depths beneath the sediment-water interface. This requires very low sedimentation rates, normal salinity and pH of the ambient seawater, and suboxic conditions where both Fe3+ and Fe2+ are thermodynamically stable. The oxidation of organic matter in peloids (particularly fecal pellets) and invertebrates may help push the oxidation state of Fe towards the 2+ state. Note that soluble Fe2+ will oxidize rapidly to relatively insoluble Fe3+ if exposed to normal, oxygenated, shelf sea water, forming compounds like limonite and goethite. Modern glauconite commonly accumulates at depths <300-500m on siliciclastic and carbonate platforms, shelves, and continental slopes. Ancient accumulations are frequently encountered as greensands.
The most common granular form of glauconite is sand- and silt-sized peloids. Other grain morphologies include vermiform (worm-like), tabular, mammillated, and composite types (e.g. Triplehorn, 1966). Glauconite can also replace or infill the chambers of bioclasts like gastropods, forams, bryozoa, corals, and the perforations in echinoid plates and spines. In hand specimen, they usually exhibit as dark green to black, sub-millimetre, ovoid pellets.
Accumulations of glauconite in the rock record are of great value because:
- They indicate a unique set of paleoenvironmental conditions.
- They are excellent indicators of stratigraphic condensation during transgression (look for a maximum flood surface at the top of the condensed section).
- They may coexist with phosphate nodules and phosphatized fossils (that also indicate condensed stratigraphy).
- Although they form authigenically, glauconite can be reworked locally. Glauconite can also be moved to deeper waters by sediment gravity flows. It is soft, friable, and has a hardness of 2 on the Moh scale, matching that of gypsum. Thus, it rarely survives beyond the first cycle of sedimentation.
Glauconite and glaucony
The name glaucony was introduced by Odin and Letolle (1980) for all forms of glauconite characterized by smectite clay crystal structure; the term glauconite was reserved for the mica-structured mineral end-member. However, the name ‘glauconite’ is firmly entrenched in decades of literature and for better or worse, I have continued with this literary laziness.
Glauconite (glaucony) in thin section
Chatham Rise
We begin with some glauconite-rich deposits from Chatham Rise, a ridge that is part of the submarine extension of Zelandia continent. The deposits form part of a highly condensed stratigraphic package dating back to the Oligocene and have been interpreted as palimpsest (relict) remnants of glacially lowered sea levels. Glauconite peloids that presently carpet the sea floor atop Chatham Rise have been K-Ar dated at 5-6 million years old (Nelson et al., 2021).
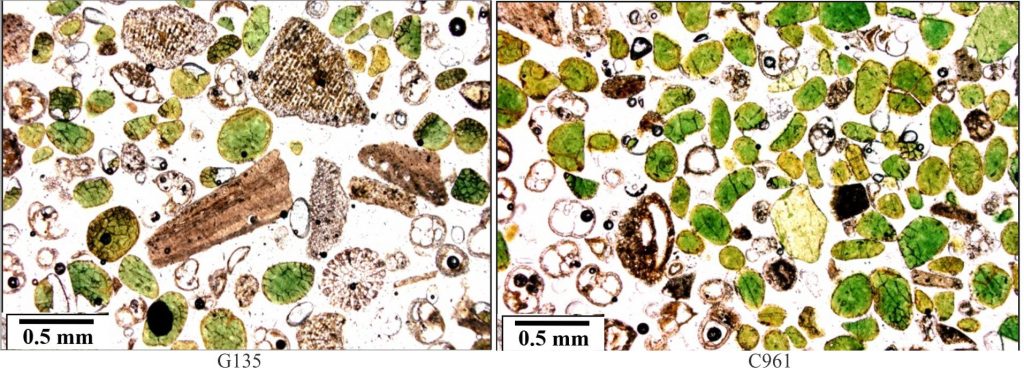
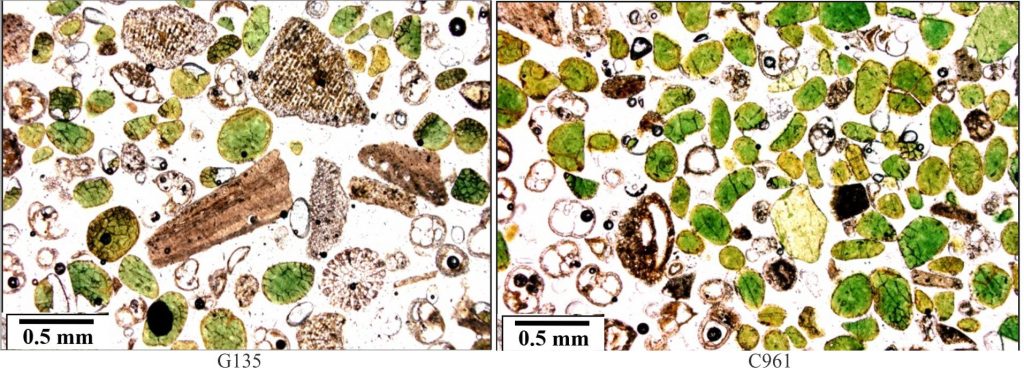
Two samples from Chatham Rise. The dominant morphology of glauconite is peloidal, with a few composite aggregates and some fossil infills (mainly benthic and planktic forams). The range of colours, from yellow-brown to grass green reflects variation in their chemical maturity – darker greens are higher in K (an average 7.2% K) and have greater crystallographic ordering determined from X-ray diffractometry. Those tending towards more brown hues have higher Fe2O3. Many peloids have a brown rim of limonite (Fe2O3). Individual peloids may also show internal colour gradation from lighter green or brown exteriors to darker green interiors. Both images plain polarized light. Images courtesy of Cam Nelson.
– Peloids are ovoid to tabular with smooth surfaces.
– Most peloids have quasi-radial or interconnected networks of cracks (dark brown – Fe oxide?). This is a common feature that mechanically weakens the grains such that they are unlikely to survive vigorous reworking or abrasion.
– Both examples contain planktic and benthic forams that show the beginnings of Fe-oxide-glauconite chamber linings.
– Image left contains fragmented echinoderm plates and spine cross-sections where perforations are partly filled with red-brown Fe oxide-glauconite.
Modern sea floor, Three Kings Islands, northern New Zealand
The shallow, modern sea floor around Three Kings Islands is accumulating bioclastic sediment, dominated by bryozoa, forams, echinoids, barnacles, and molluscs. In this mix are glauconite pellets and glauconite infilled bioclasts.
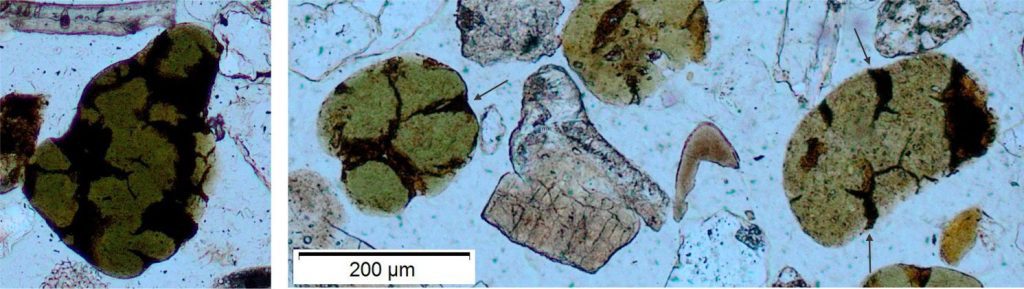
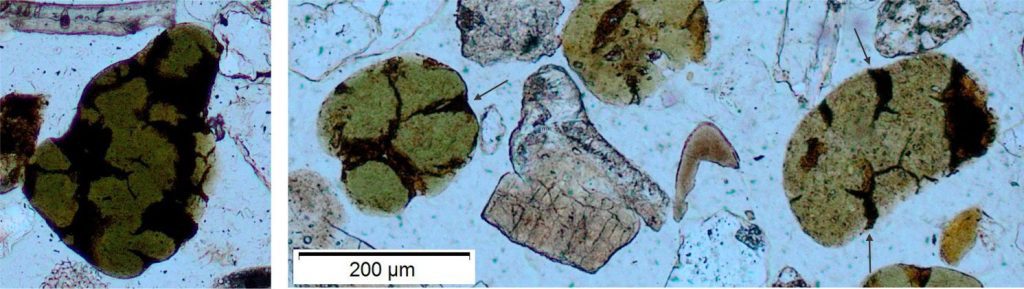
Left: An irregular peloid with cracked, vermiform glauconite. The dark brown-black, opaque (and isotropic) material, that along its edges is yellow-brown, may be limonite. Right: Peloidal glauconite in shades of green-brown and yellow-brown. The cracks typically taper towards the peloid centre (arrows). The cracks may have formed by syneresis (a process of fluid expulsion typically associated with the transformation of gels to crystalline materials – I think the jury is still out on this interpretation). Cracks will render a peloid more vulnerable to disintegration from vigorous reworking; thus, the present examples have probably moved very little. Both images in plain polarized light. The bar scale applies to both images.
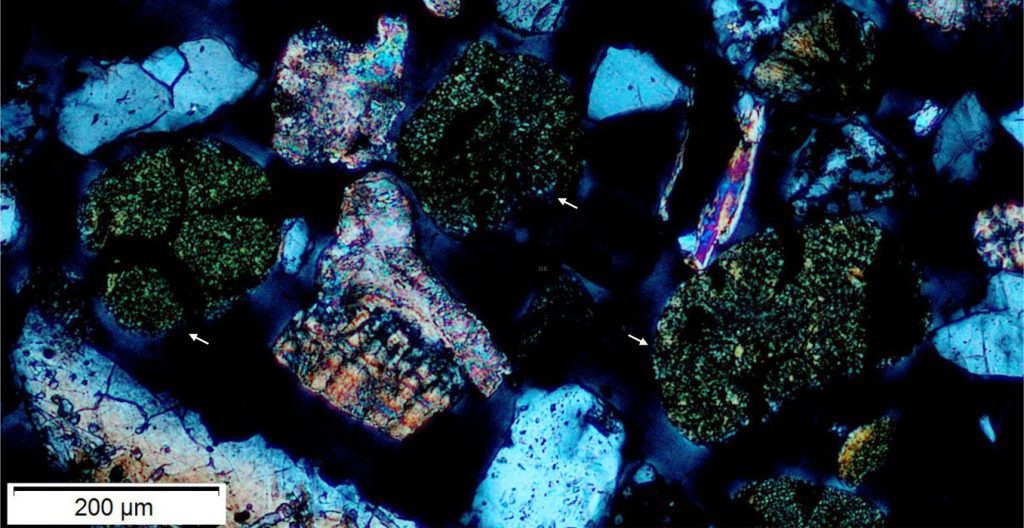
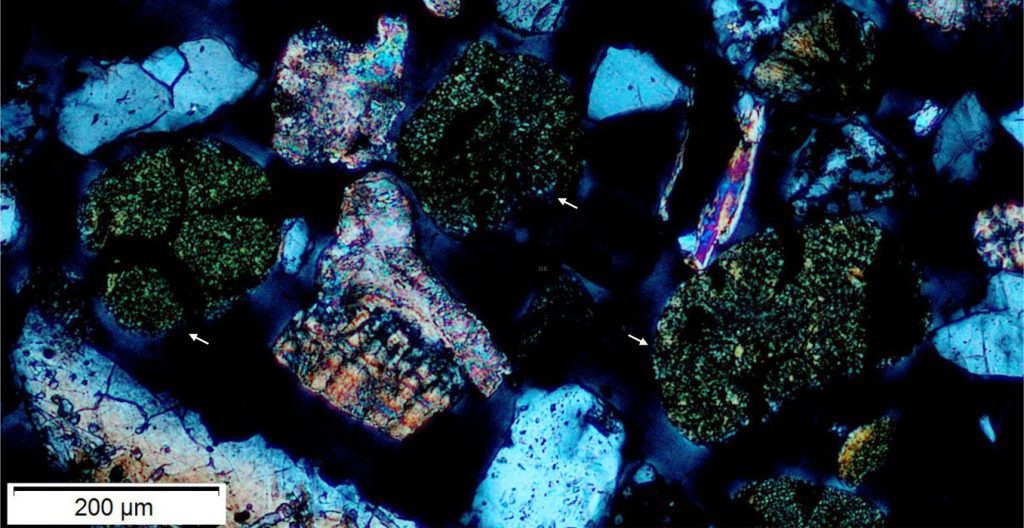
The microcrystalline habit of glauconite presents as a speckled appearance, best seen under crossed polars. The three grains (arrows) are the same as in the plain polarized image above. Grains with first-order grey interference colours are quartz. The bioclasts are probably molluscs and barnacles.
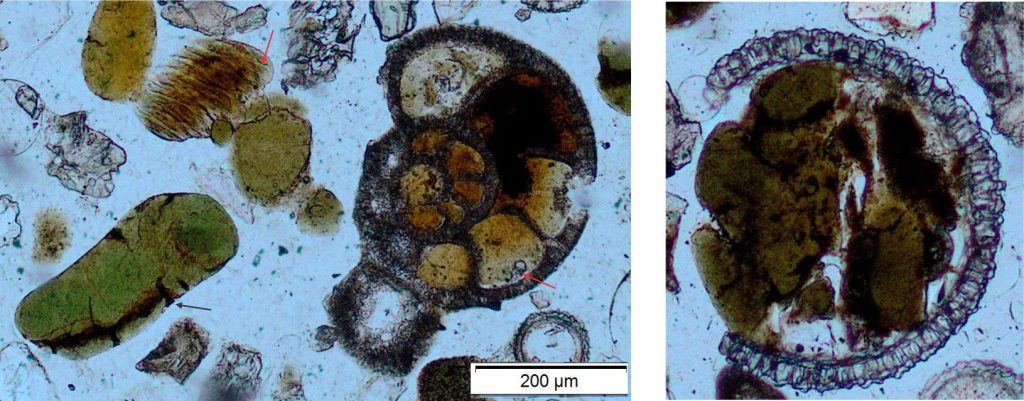
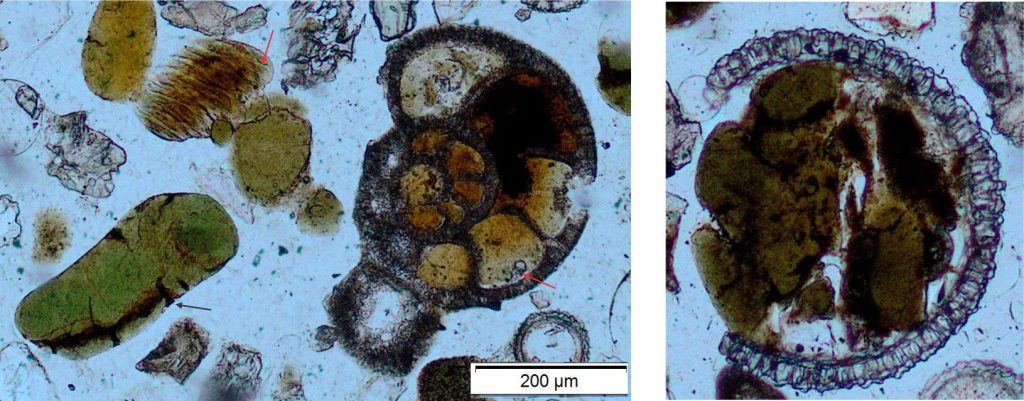
Left: Three things to note here: 1. The planispiral foram chambers have incipient glauconite fill, that along the inner margin of the test wall are transforming to pale green (red arrow). The radial crystallite wall structure is still visible. 2. Three ovoid glauconite peloids to the left of the foram display variations in colour that probably correspond with a range of maturities. The larger grain has developed a few cracks (black arrow). 3. The grain top left resembles barnacle plications; here too glauconite has begun to form with the outer grain rim developing green hues (arrow). Plain polarized light.
Right: A rotaliid foram chamber (radial wall structure) partly filled by yellow-green glauconite and a few silt-sized fragments of quartz. The left side of the fill shows mammillated morphology. Plain polarized light.
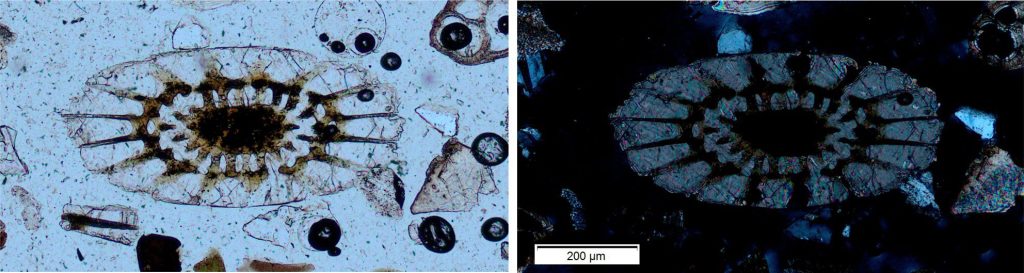
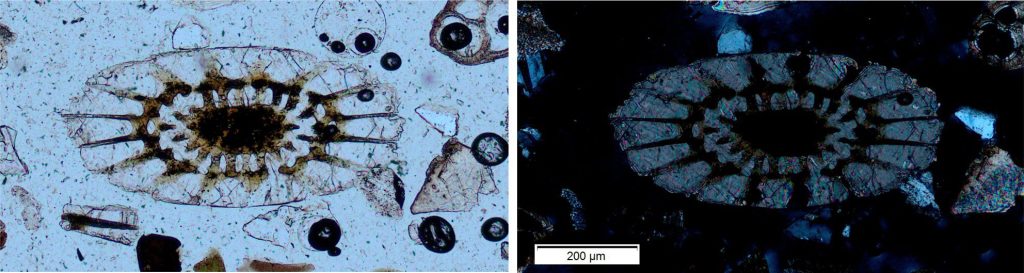
An oblique section through an echinoderm spine where the internal perforations are filled with immature, brownish glauconite. Left – plain polarized light. Right – crossed polars. The bar scale applies to both images.
Glauconite infill of fossils
Examples from Oligocene bioclastic limestones, Te Kuiti Group, New Zealand
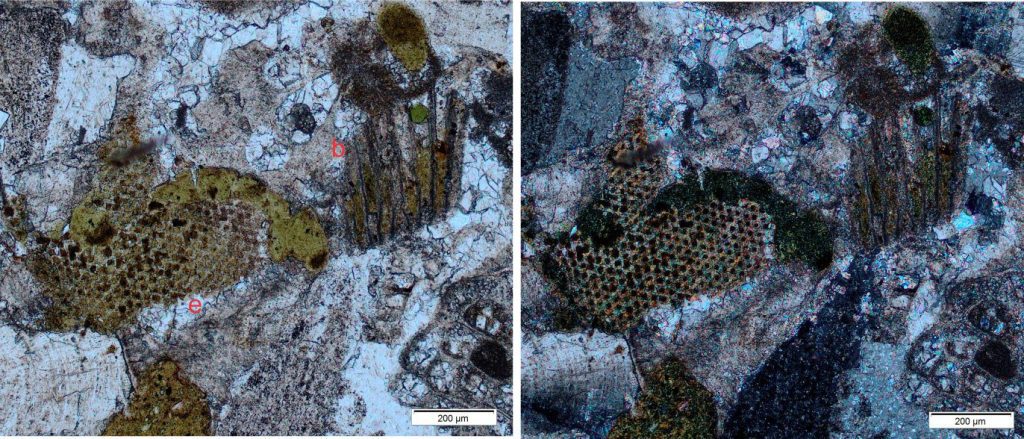
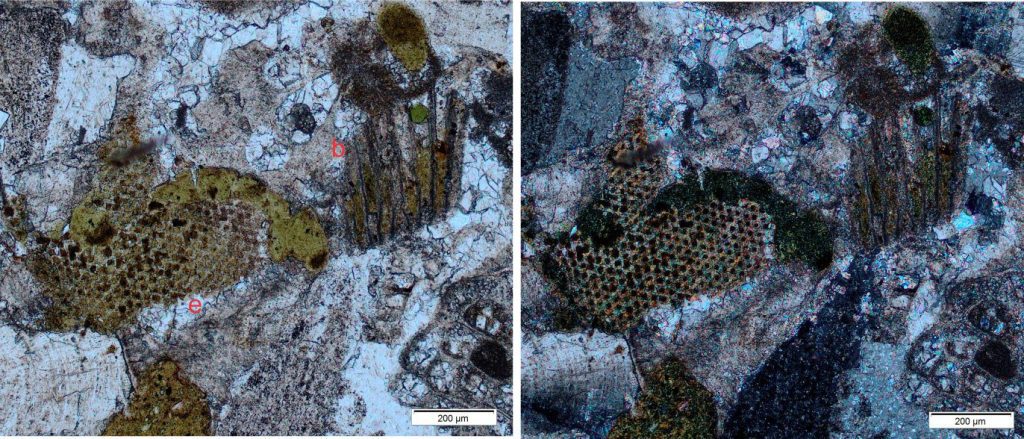
The emphasis in this image pair is glauconite filling invertebrate microporosity: on the left the perforations in an echinoderm plate (e); at centre-right a longitudinal section through bryozoa zooids (b). An irregular, composite mass of glauconite has also formed along the echinoid plate margins also contains silt-sized quartz grains. The bioclasts are cemented by coarse calcite spar. Left – plain polarized light; Right – crossed polars.
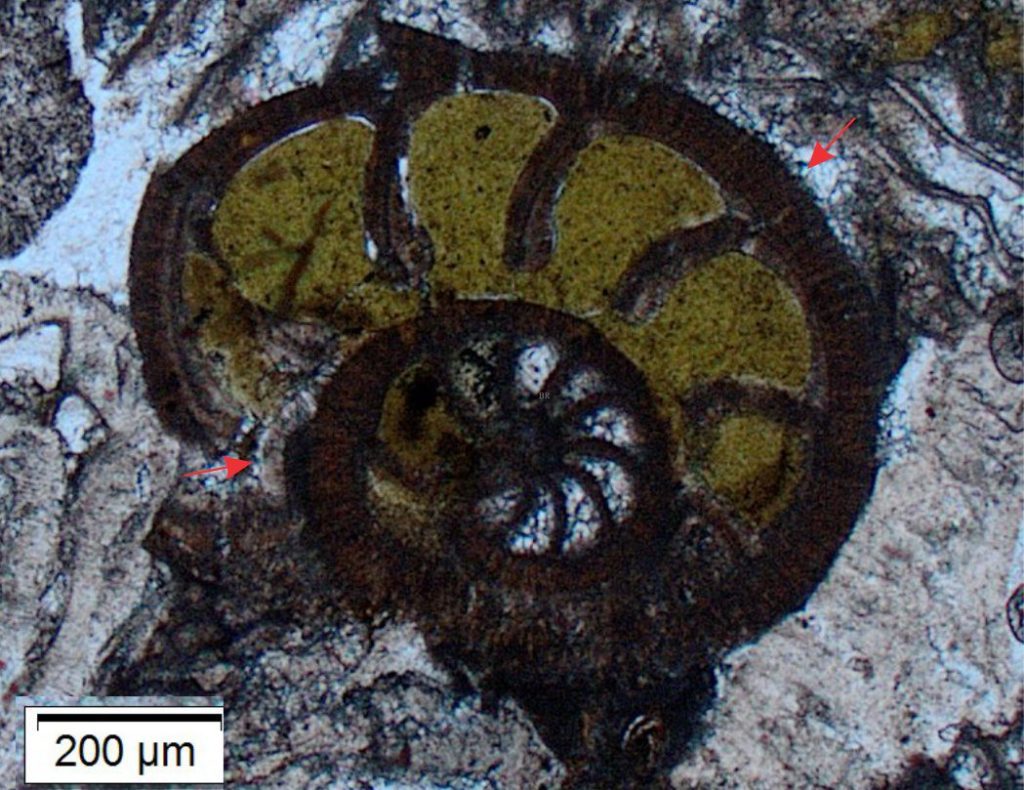
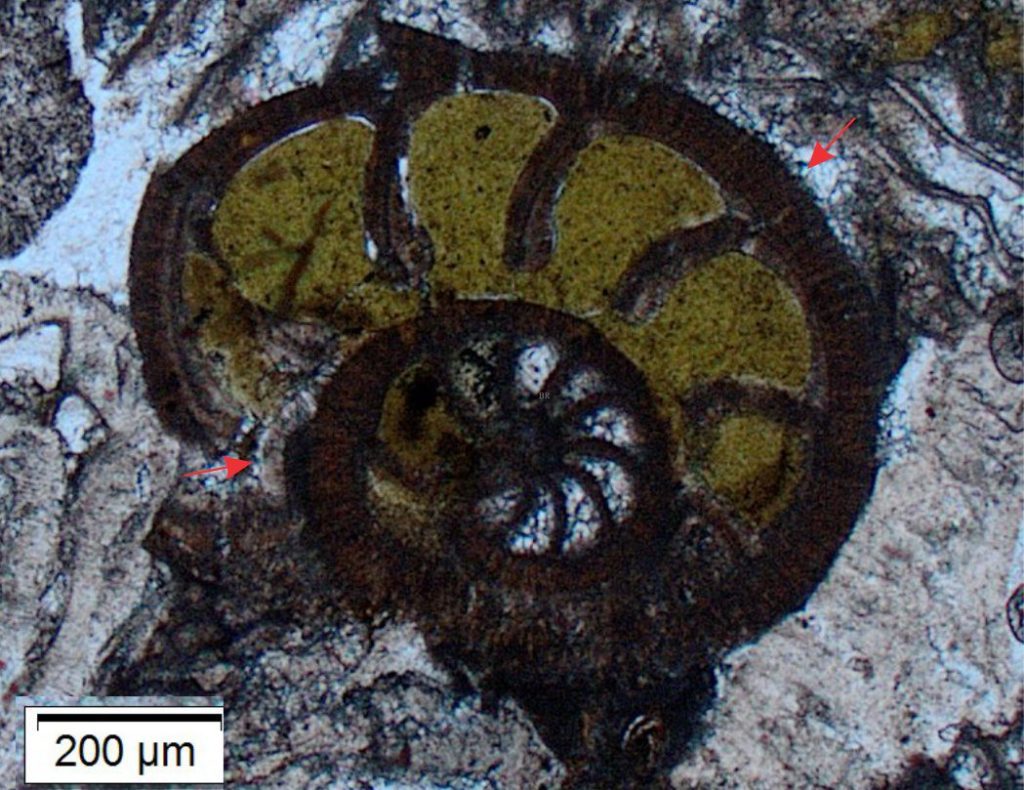
The outer chambers of this rotaliid foram are filled with yellow-green glauconite; the inner chambers with calcite spar. The radial wall structure is still visible although much of the calcite has been replaced by limonite and glauconite. The outer margins of the foram may have an isopachous calcite rind (arrows). The remainder of the cements are coarse calcite spar. Plain polarized light.
Glauconitic micaceous arenite
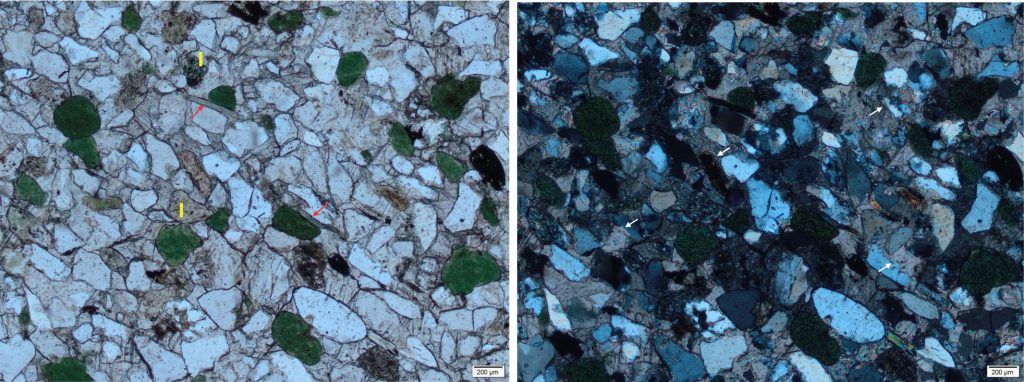
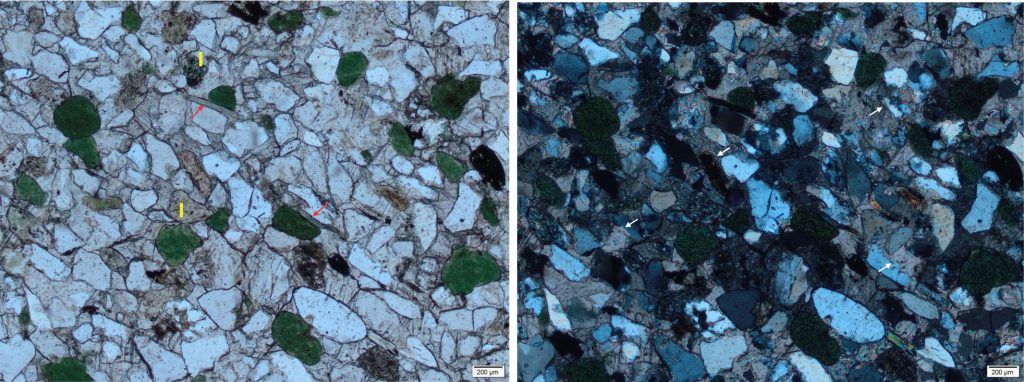
– Glauconite peloids up to 0.5mm across (10%). Some glauconite grains are deformed around stronger quartz and feldspar grains (by compaction).
– Quartz grains (70-75%) are mostly monocrystalline (there are a few polycrystalline grains) and show a mix of unstrained and strained extinction. Grains are angular to well rounded, but some of the angularity is due to calcite replacement of quartz (some examples shown by white arrows).
– Untwinned K-feldspars (5-10%) show varying degrees of sericite alteration. Some appear superficially like lithic grains.
– Lithics (I) (~5-8%). Be careful to distinguish actual lithics from altered feldspar grains.
– White micas (1-2% – red arrows) show some alteration to chlorite (bluish interference colours).
– The framework clasts and micas have a crude alignment along the top left/bottom right diagonal.
– Cement is almost entirely coarse calcite. Contact between the calcite cement and framework grains is irregular, indicating silica replacement.
Glauconitic arenite
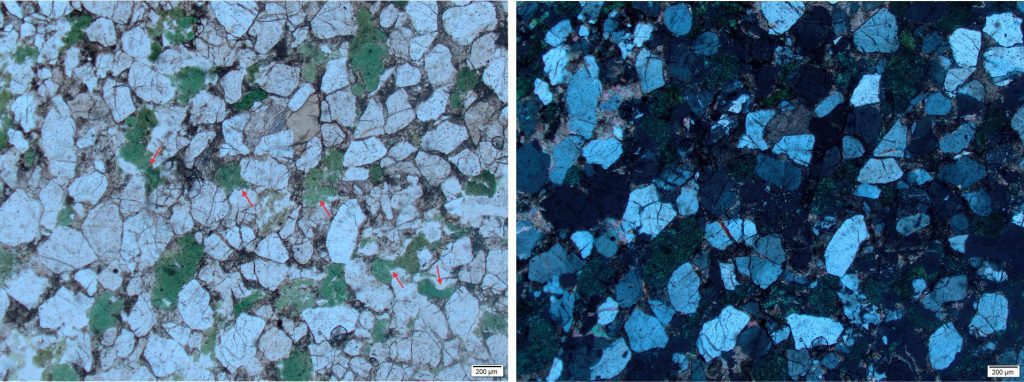
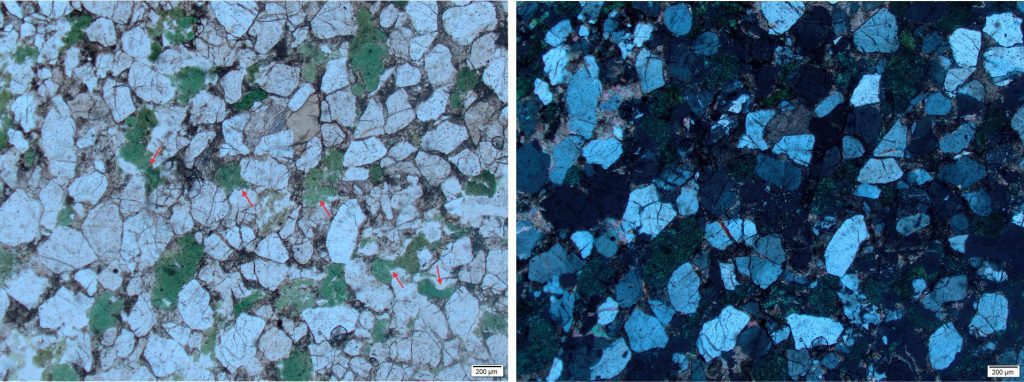
The sandstone framework here is compositionally similar to the arenite above, but note the changes in texture and fabric:
– The grains are more densely packed, with significantly less calcite cement and decreased calcite replacement of framework grains.
– The original peloidal shape of the glauconite grains has been distorted by compaction around stronger quartz-feldspar and squeezed between some grains (red arrows for some examples).
– Compaction has fractured some quartz grains – fractures are filled by calcite and possibly clays.
Other posts in this series
Brachiopod morphology for sedimentologists
Bivalve shell morphology for sedimentologists
Gastropod shell morphology for sedimentologists
Cephalopod morphology for sedimentologists
Optical mineralogy: Some terminology
Carbonates in thin section: Forams and Sponges
Carbonates in thin section: Bryozoans
Carbonates in thin section: Echinoderms and barnacles
Carbonates in thin section: Molluscan bioclasts
Neomorphic textures in thin section
Lithic grains in thin section – avoiding ambiguity
Mineralogy of carbonates; skeletal grains
Mineralogy of carbonates; non-skeletal grains
Mineralogy of carbonates; lime mud
Mineralogy of carbonates; classification
Mineralogy of carbonates; carbonate factories
Mineralogy of carbonates; basic geochemistry
Mineralogy of carbonates; cements
Mineralogy of carbonates; sea floor diagenesis
Mineralogy of carbonates; Beachrock
Mineralogy of carbonates; deep sea diagenesis
Mineralogy of carbonates; meteoric hydrogeology
Mineralogy of carbonates; Karst
Mineralogy of carbonates; Burial diagenesis
Mineralogy of carbonates; Neomorphism
Mineralogy of carbonates; Pressure solution
Mineralogy of carbonates: Stromatolite reefs

















