









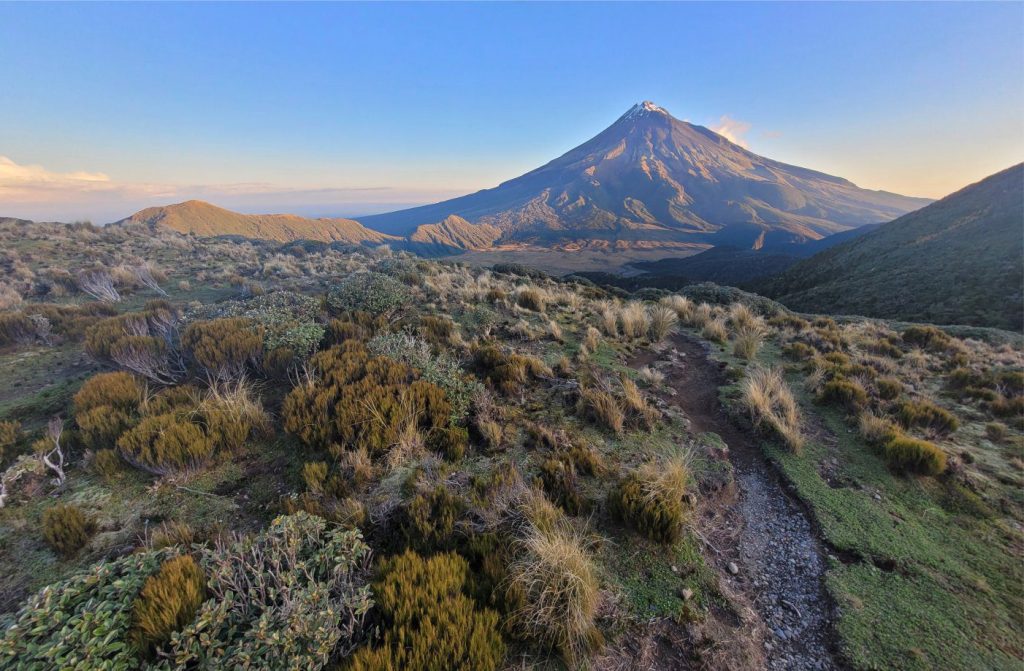
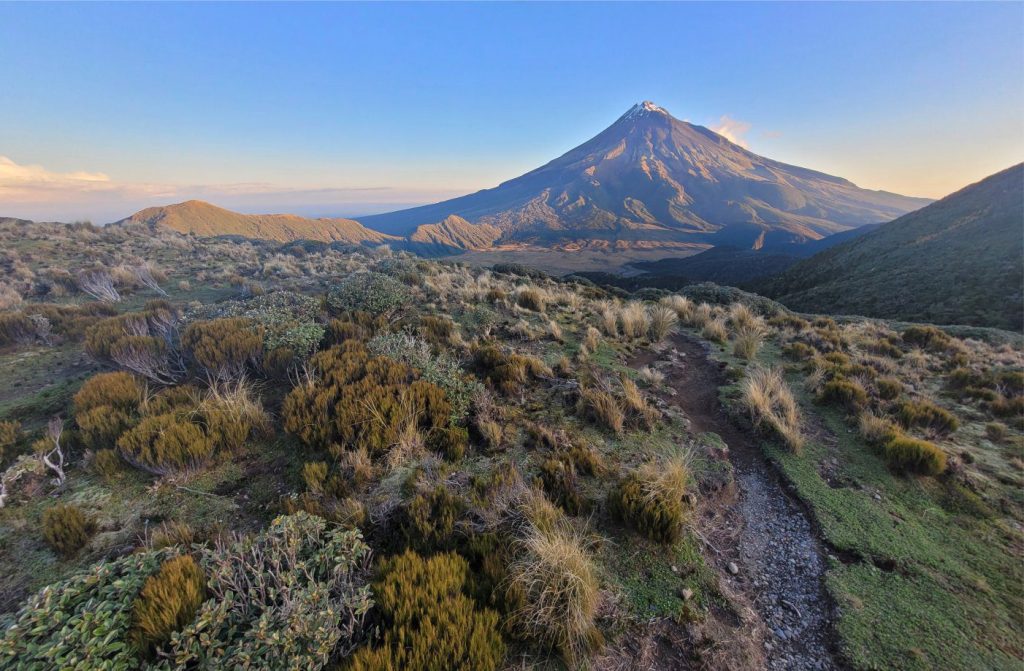
The almost perfectly symmetrical profile of the Late Pleistocene stratovolcano Mt. Taranaki, the late afternoon sun emphasizing its ribbed carapace and incised mountain streams. The view is from Pouakai Track, where there is an overnight camp to complete the end of a fine day’s hike from the mountain flank. Hiked with my son Sam Ricketts, who took this photo.
Mountain streams – the ultimate source of sediment for many fluvial systems
The idea for writing this brief arose whilst struggling through some challenging trails on Mt. Taranaki (with son Sam – I was struggling, he wasn’t), a dormant stratovolcano that is responsible for the prominent topographic salient on North Island (NZ) west coast.
Published records of ancient fluvial depositional systems are replete with examples of mud, silt, sand, and gravel that are organized into lithofacies and assemblages of lithofacies. The important word here is “organized“:
– we recognise the physicality of an organized world, for any science, but in this case of sediments, sedimentary structures, and stratigraphic trends.
– Our methodological attachment to “organization” grants us a warrant to interpret this world and, at least in our own minds, create order out of apparent disorder.
The degree of organization we commonly observe in fluvial deposits (witness all those lovely models) belies their origins. Most fluvial drainage systems begin on steep slopes where water flow is usually confined to single, steep-sided, boulder-strewn channels (some rivers spill from lakes or springs). Flow is frequently torrential, but just as commonly reduces to a trickle – there is no significant base-flow that, in most low-gradient rivers, helps sustain the supply of water to channels when there is no overland flow. The bedforms that help us identify the different components of fluvial systems are absent or rare in mountain streams.
Despite their importance, mountain streams are rarely incorporated into fluvial models. At best, the models make brief reference to some distant source. I suspect this discrepancy is a function of their preservation potential rather than neglect. Mountain streams are entirely dependent on some pre-existing, steep, hill-slope topography. Confident recognition of mountain streams in the rock record would require identification of this paleotopography. There may be some relief from this dilemma where steep topography is associated with faults, for example in rift basins, or basins formed at the releasing bends of strike-slip faults. The Violin Breccia in Ridge Basin, California, may contain possible examples of this type of preservation (e.g., Crowell, 2003; Link and Crowell, 2003). Cretaceous, synrift, chaotic breccias in the Cauvery rift basin, SE India, have also been interpreted as base-of-slope talus fans associated with normal faults, that may also qualify as steep gradient stream-fed deposits (Chakraborty and Sarkar, 2018; Open Access).
The following notes focus on mountain streams but should apply to any steep-slope, coarse bedload channels.
Channel characteristics
Geomorphologists generally consider grades of 3% to be steep, but mountain streams can have average grades greater than 20%. Steep gradient channels are commonly interrupted by pools, boulder steps, and bedrock waterfalls. Channel gradients are therefore expressed as averages.
Channel widths are usually measured in metres. Under conditions of normal flow, water depths are less than the diameter of the largest boulders.
Mountain stream channels are commonly floored by bedrock. However, mountains continuously shed eroded and weathered rock fragments, and in the case of volcanic edifices this includes airfall debris (ash, lapilli, blocks) and the deposits of pyroclastic density currents. Streams that flow across this colluvium and airfall mantle will erode through it rapidly until they reach bedrock. Thus, stream banks and valley walls tend to be steep. Failure of slopes underlain by colluvium can change the course of channels as well as delivering important sources of clastic sediment.
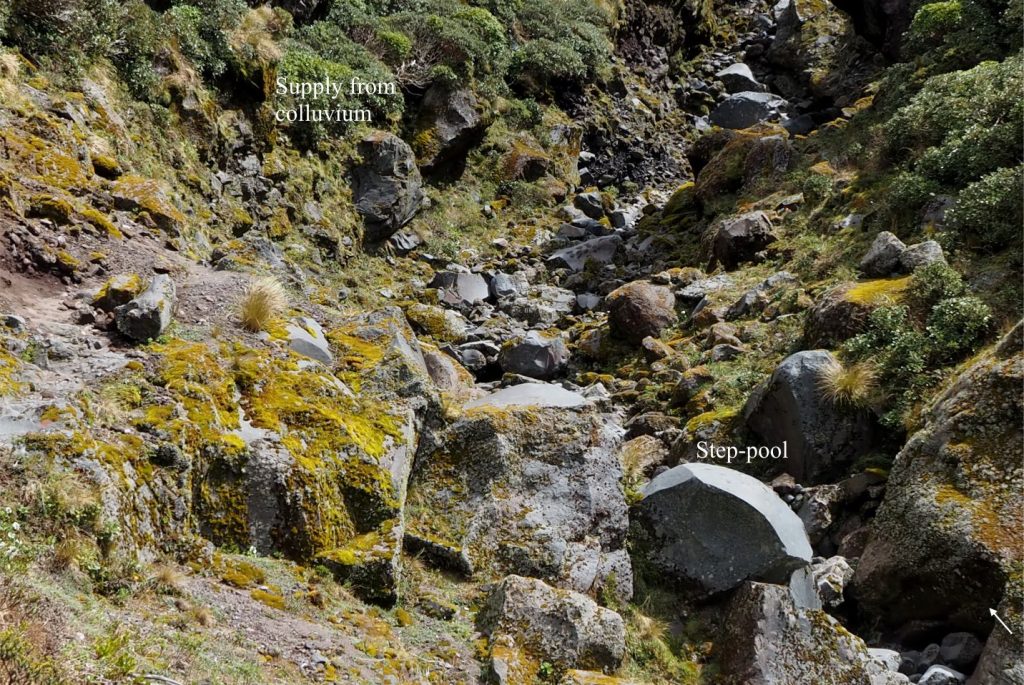
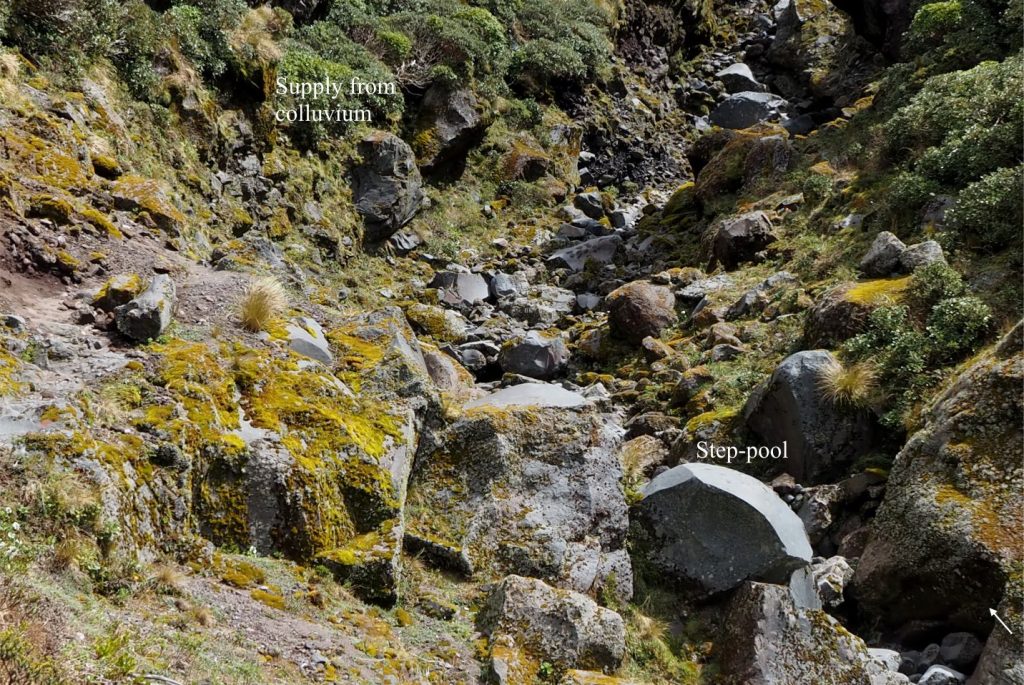
A narrow, boulder channel with blocks of andesite to 4 m – the block indicated by the arrow is about 2 m long dimension. Summer flow is reduced to a trickle. The main source for these angular, disorganized blocks is a jointed andesite flow about 100 m upstream. Some clasts are also derived from the boulder colluvium exposed along the channel margins. Holly Hut track, Mt. Taranaki, New Zealand.
A common feature of mountain streams is the interruption of channel flow by pools, steps, and riffles arranged in staircase-like successions. Steps form at breaks in bedrock, or where boulders (and logs) are jammed across the width of a channel, creating temporary barriers to flow. Water will flow over the top of the steps during high precipitation or snow melt but will flow through the interstices between packed clasts during periods of low water. Pools develop on the upstream side of the steps. Riffles also alter channel flow but in this case the structure is a bar-like accumulation of gravel, such that flow tends to increase across the shoaling upstream slope but decrease on the downstream face of the gravel bar.
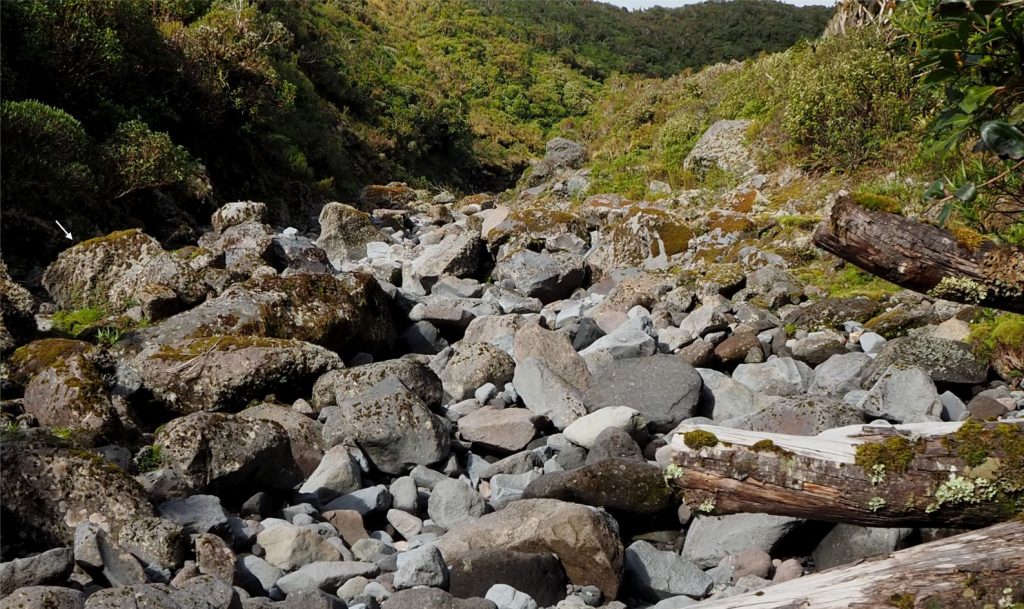
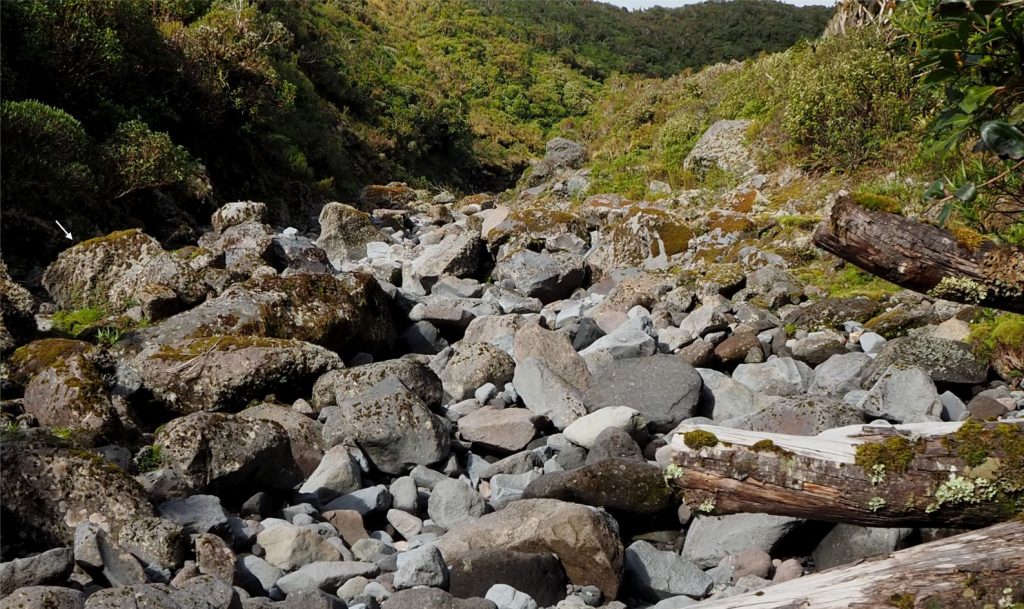
This boulder filled channel occurs on slightly lower gradient slopes about 2 km from the primary source of andesite flows. There is some evidence of clast rounding, although sorting is extremely poor. Water flow in this view is directed through large pore spaces in the gravel framework that exits into surface pools. The block indicated by the arrow is 1.5 m long dimension. Holly Hut track, Mt. Taranaki, New Zealand.
Flow characteristics
Flow velocities are highly variable because of pool-steps and riffles, boulders and logs that commonly litter channel floors, and the general bed roughness created by variable clast sizes. Flow rates as high as 5-8 m/s have been recorded. Flow can change abruptly from subcritical to supercritical both across a channel, and in the downstream direction. Supercritical flow over a riffle or boulder step rapidly changes to subcritical flow in the downstream pool where sand and finer grained sediment is deposited. The turbulence of hydraulic jumps at this transition can erode loose sediment at the base of the step, resulting in partial collapse or rolling of large clasts. Turbulence is also generated in plunge pools below larger boulders or bedrock walls. Flow over submerged boulders can produce stationary waves (standing waves), particularly during floods. Large, immovable boulders also play an important role in reducing flow energy because of frictional drag.
Flow velocities, even during floods, may not be sufficient to move some of the largest boulders, which begs the question – how did they get there? Three possible mechanisms are:
- Large blocks have broken or toppled from a steep bedrock face, or from colluvium exposed on the adjacent channel margin, and rolled downslope.
- The blocks were emplaced by debris flows or lahars, the subsequent stream flow removing the finer-grained matrix.
- On volcanic slopes, large ballistic blocks can be embedded in finer ash. If the softer ash is subsequently eroded, the blocks will join the channel bedload.
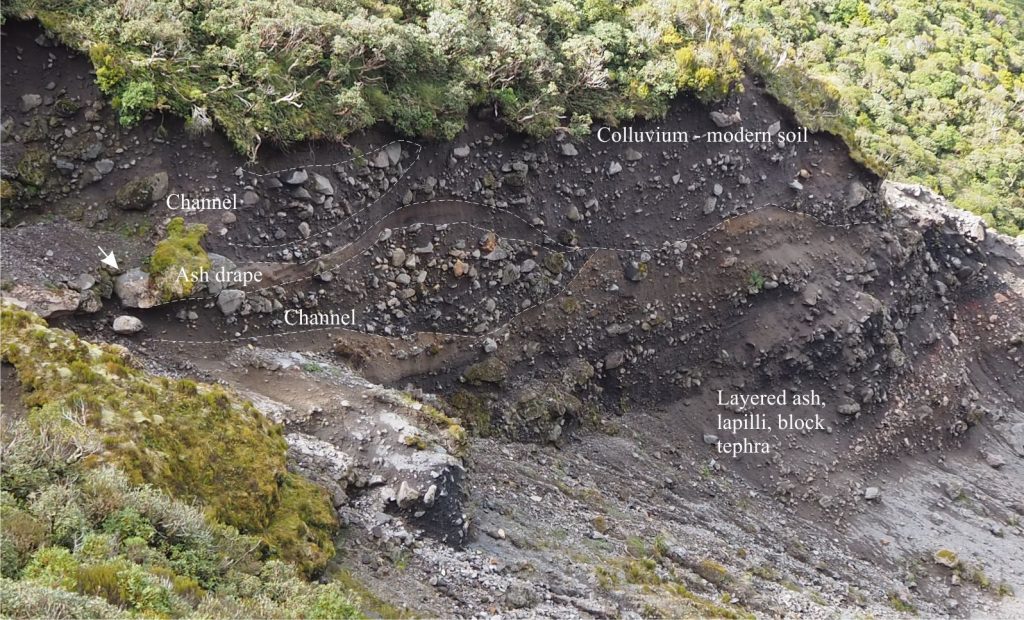
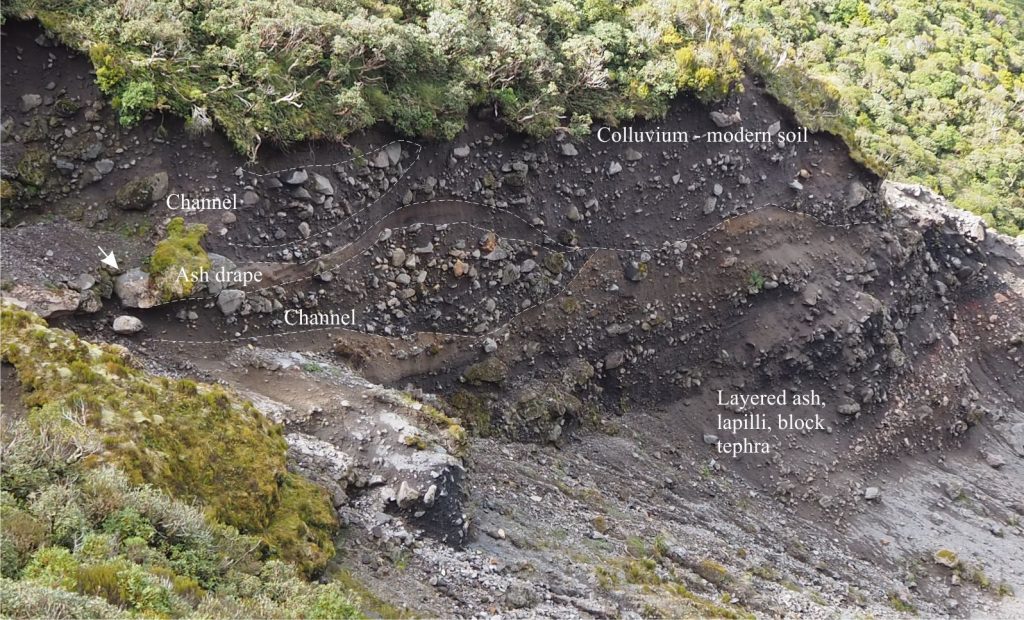
Colluvium exposed in a slump scarp on Mt. Taranaki stratovolcano contains two former channels (outlined) filled with a mix of poorly sorted gravel (clasts of andesite) ash and lapilli. The lower channel cuts into bedded ash-lapilli tephra that also contain some larger blocks (probably ballistics). Both channels are draped by airfall tephra layers. The boulder at left-centre is about a metre across (arrow). Holly Hut track, Mt. Taranaki, New Zealand.
Textural characteristics
The most obvious gravel components in mountain streams are cobbles and boulders. However, finer grained sediment is nearly always present – pebbles, sand, silt, and their volcaniclastic grain size equivalents are deposited in pools and in the lee of larger clasts. Thus, clast sizes along any channel reach can range over 3 or 4 orders of magnitude, from silt to boulders a metre and more in diameter. Sorting is at the extreme end or ‘poor’, with standard deviations ranging over 8-12 phi. Even the pockets of sand are poorly sorted.
Most gravel clasts derived from bedrock begin life in angular mode, although a degree of rounding can occur surprisingly rapidly within a few 100 m of transport downstream. Even large, immovable boulders develop some rounding on their upstream faces because of the high number of impacts during floods.
Streams that erode older sedimentary rock, particularly conglomerate, will potentially inherit some of the pre-existing textural properties. Thus, beds of older conglomerate that consist of rounded clasts may release their clasts intact. Likewise, joints and fractures in bedrock and lava flows will dictate the initial shape of clasts.
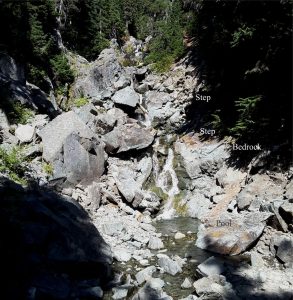
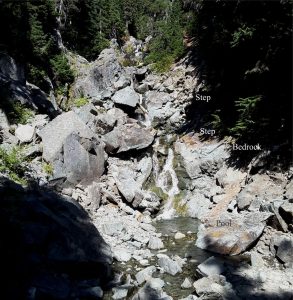
Angular, boulders, cobbles and pebbles of intensely fractured granodiorite overlying a channel base of the same rock type. The step in the foreground consists of bedrock and fitted boulders. Boulder orientation is haphazard. The larger boulders dampen flow during flood events and trap smaller cobble and pebble-sized clasts. Mt. Garibaldi trail, southern British Columbia.
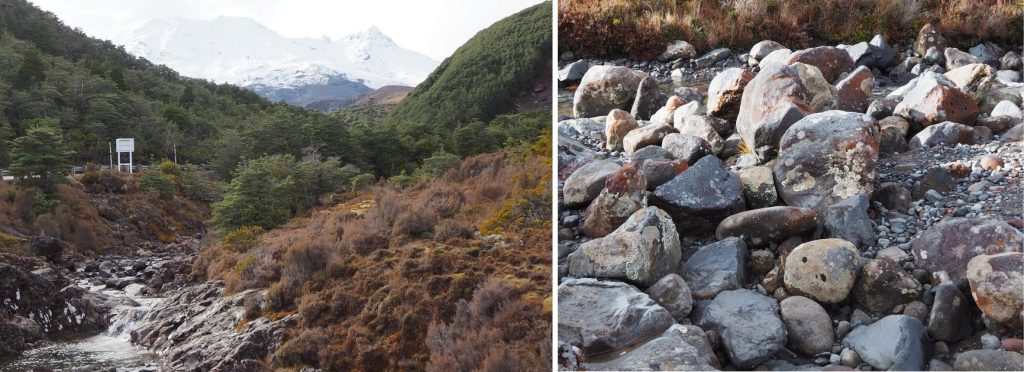
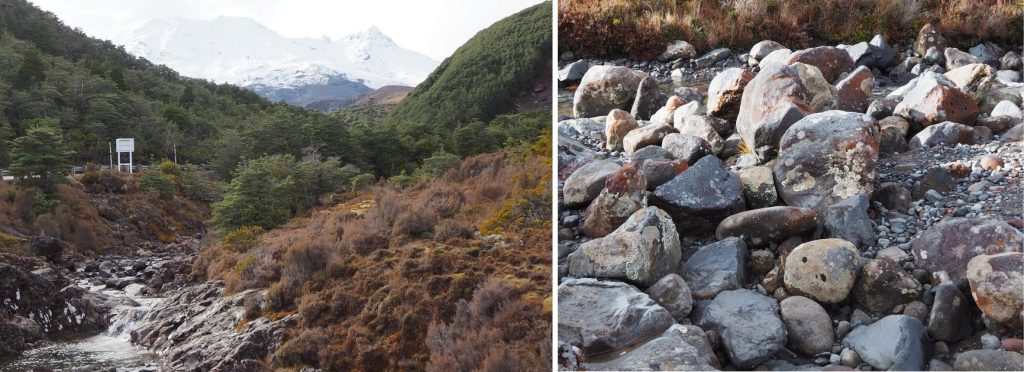
Left: Bedrock floored boulder stream draining the outer slopes of the active volcano, Mt. Ruapehu, New Zealand. There are multiple bedrock and boulder steps and pools along its course. The channel margins are mantled by coarse- and fine-grained tephra, colluvium, and paleosols. Right: An exposed part of the active channel containing a mix of rounded and angular gravel clasts (andesite and basalt) and scattered pockets of sand. The summit is about 5 km away (line of sight), along which there are multiple bedrock (lava flows), tephra, and colluvium sources. Significant rounding of clasts can occur over that distance.
Sources of gravel
Mountain uplift is always accompanied by weathering and erosion of exposed bedrock – a continuous battle among competing processes and forces (tectonic uplift, denudation, isostatic adjustment). On the denudation side, fragmental debris is produced by the elements of weathering (e.g., precipitation, freeze-thaw, soil formation), and the forces of gravity, the latter witnessed as rock falls and topples, landslides, sector collapses, soil creep, and debris flows. Active volcanoes add all the products of airfall and pyroclastic density currents to this mix.
Some of this debris is added directly to existing mountain streams. However, most of the debris accumulates as colluvium on the mountain or steep hill-slope flanks, where it remains in storage until it is released to active stream beds. Depending on the local climate, soils formed over the colluvium will help stabilize this sediment mantle; the soils are a potential source of muddy sediment and a cause of mineral instability, dissolution, or replacement. Steep slope colluvium is frequently subjected to failure that can:
- Supply fine and coarse-grained sediment to existing channels.
- Focus overland flow and the development of new channels.
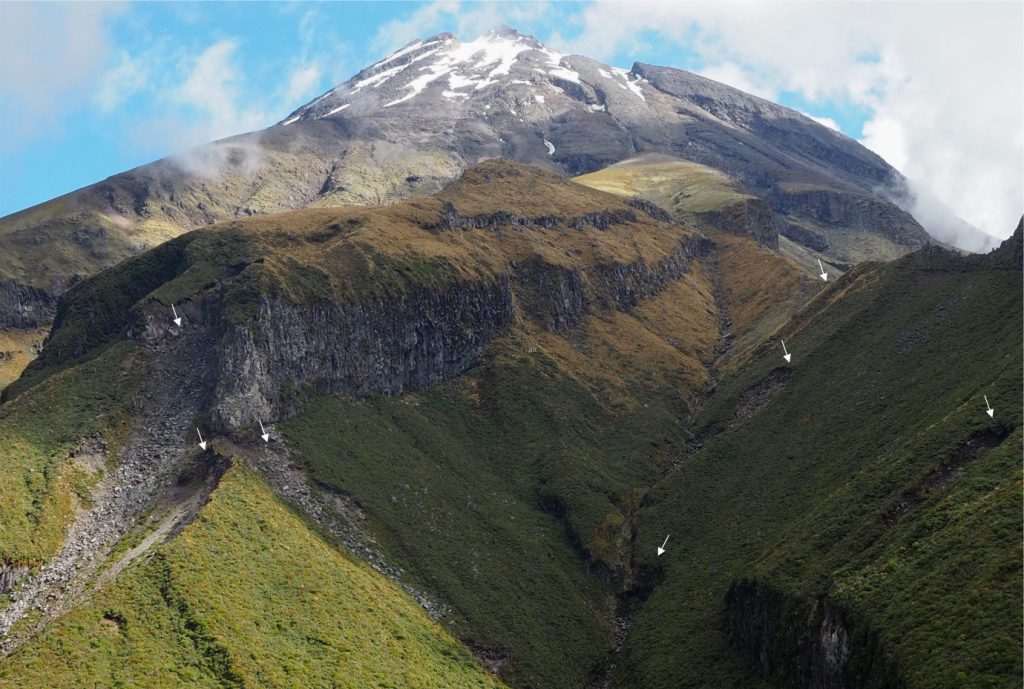
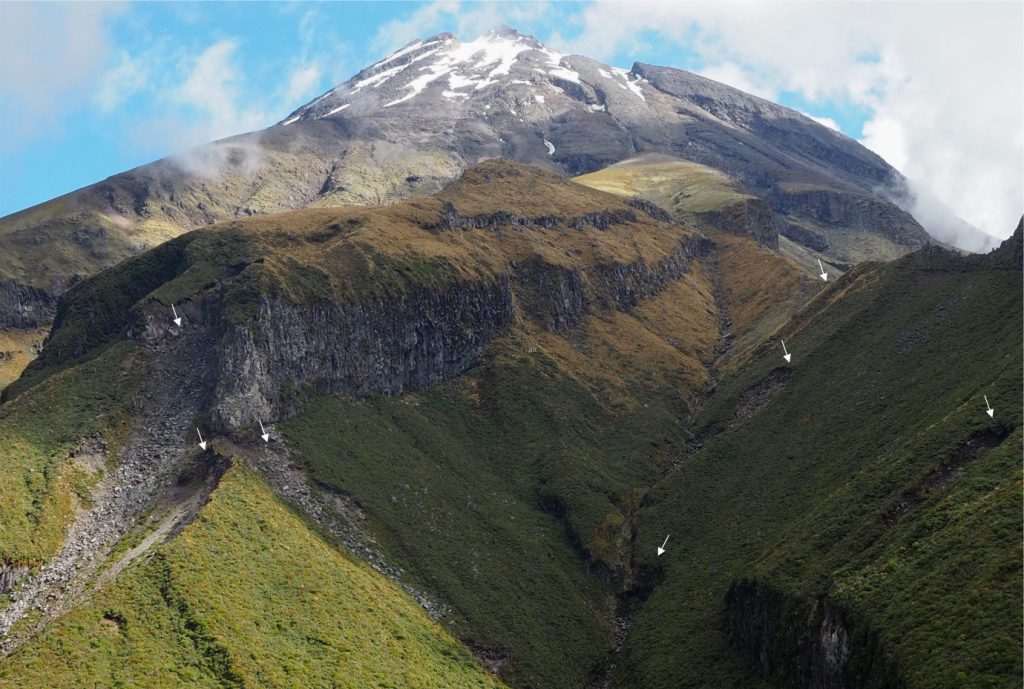
Bluffs that expose stacked, columnar jointed lava flows are a prime source of blocky fragments that tumble or slide directly into the narrow channel or become part of the slope colluvium. Supply of debris to the channel also occurs via gravitational failure of the colluvium – seven recent failures and their head scarps are visible here (arrows). The main bluff (left-centre) is about 75 m high. Holly Hut track, Mt. Taranaki, New Zealand.
As depositional slopes decrease…
Ultimately, the very coarse, poorly sorted deposits of mountain streams are destined for talus fans, alluvial fans, and a diverse array of river systems, but en route there are significant changes to the nature of the channels, the character of the sediments and their lithofacies:
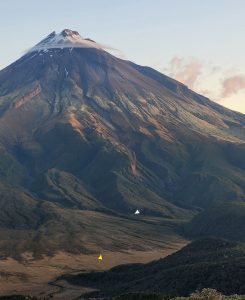
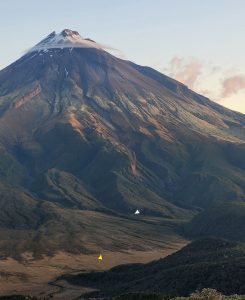
One of the many mountain streams that drain the slopes of Mt Taranaki merging into a small, lower gradient alluvial fan near the base of the edifice. The outer edge of the fan (yellow arrow) interfingers with a swamp (brown hues). Unlike the mountain torrents, the alluvial fan is characterised by gravel bars and bedforms, and an improvement in clast sorting. Holly Hut indicated by the white arrow. Pouakai track, Mt. Taranaki, New Zealand. Photo by Sam Ricketts.
- Mountain streams merge with alluvial fans, trunk, and distributive river systems. They can also flow directly into seas and lakes.
- Channels tend to develop perennial flow with the downstream addition of tributaries and base-flow.
- Base level becomes increasingly important as a determinant of channel sinuosity. Changes in base level, for example sea level, are unlikely to have much effect on steep gradient mountain streams.
- Bedload commonly changes downstream from gravel-dominated to sand-silt-mud dominated (although not always uniformly).
- The development of floodplains; not seen in mountain streams.
- The development of bedforms in sand and gravel.
- Downstream reduction in grain size. This may not be obvious where a drainage basin has multiple sediment sources and points of sediment entry along its rivers.
- Downstream changes to clast rounding and sorting – these textural properties usually improve.
- Mechanically unstable components are preferentially removed – this includes minerals possessing good cleavage, and clasts prone to breakage because of sedimentary layering (e.g., shale), penetrative cleavage, joints, or fractures.
- Hydraulic sorting and winnowing of lower specific gravity minerals. The classic example of this process is the increase in the proportion of quartz grains at the expense of lithic, feldspar, and heavy mineral grains with distance and time from the primary source.
- Potential chemical removal of less stable components (e.g., some ferromagnesian minerals, feldspars, carbonates). Development of soils on floodplains is an important part of this process, where changes in soil-water pH and REDOX conditions can affect mineral stability.
Other posts in this series
Sandstone lithofacies
Sedimentary lithofacies – An introduction
Ripple lithofacies: Ubiquitous bedforms
Ripple lithofacies influenced by tides
Tabular and trough crossbed lithofacies
Laminated sandstone lithofacies
Low-angle crossbedded sandstone
Hummocky and swaley cross-stratification
Lithofacies beyond supercritical antidunes
Subaqueous dunes influenced by tides
Gravel lithofacies
Introducing coarse-grained lithofacies
Crossbedded gravel lithofacies

















