













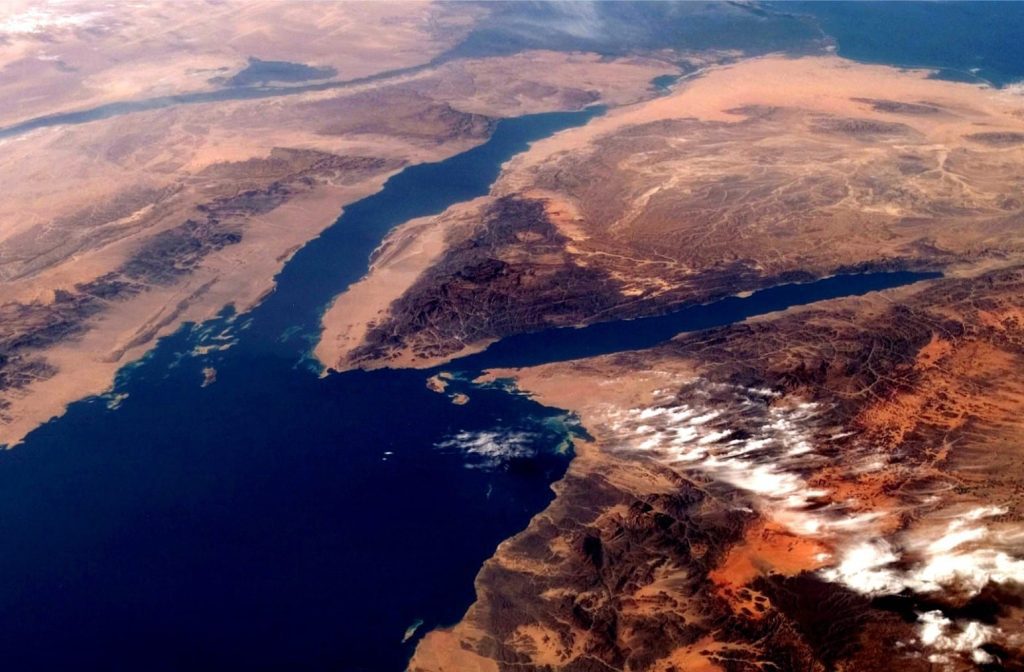
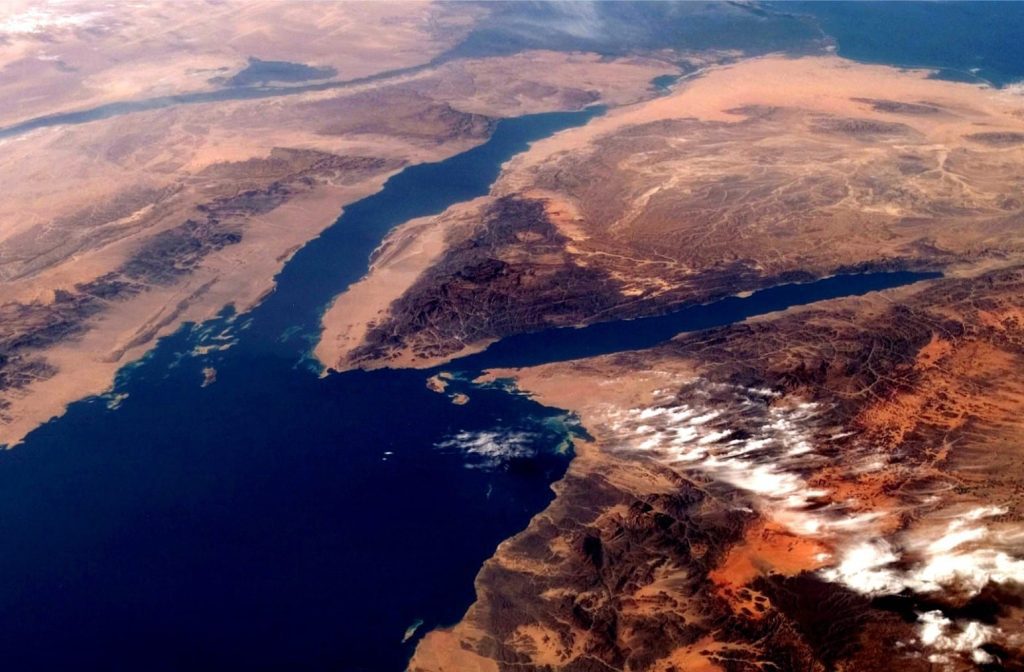
Oblique view of northern Red Sea, Gulf of Suez (top center), and Gulf of Aqaba (right). Elevated topography of the rift shoulders (dark terrain) are nicely contrasted with the adjacent plains. Nile River snakes its way across the top left; we get a glimpse of Nile delta at top center. Image credit: NASA
Progression of continental rifting to sea floor spreading creates paired (conjugate) passive margins over which post-rift successions accumulate across crust transitional between fully continental and fully oceanic.
The East African Rift System (EARS) provides one of the best actualistic demonstrations of continental rifting, from nascent domal uplift at its southernmost end, to mature, extensional faulting, volcanism, and sediment-filled grabens in the Ethiopian Rift. Afar Depression appears to be on the verge of transforming to the earliest stage of sea floor spreading.
EARS is part of a geodynamic trio: Red Sea and Gulf of Suez lie northeast, and Gulf of Aden northwest. Afar Depression is their triple junction. The Red Sea – Gulf of Aden combo provide excellent examples of nascent sea floor spreading and the transition from continental rifting to passive margin. Their present plate tectonic configurations are as follows:
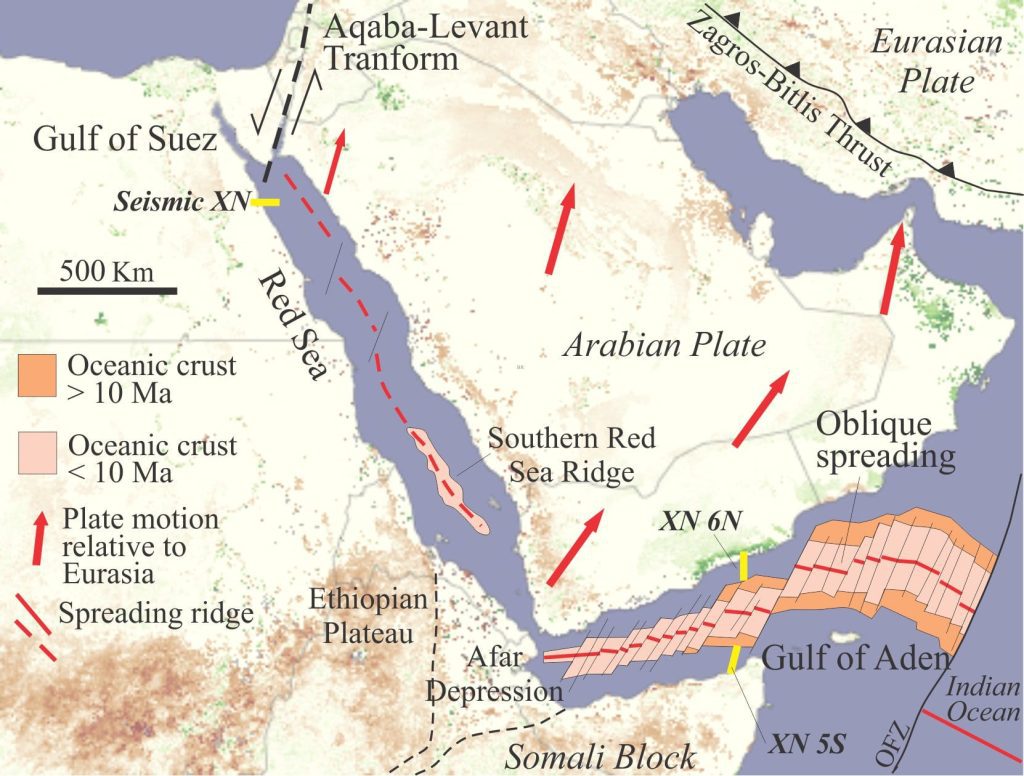
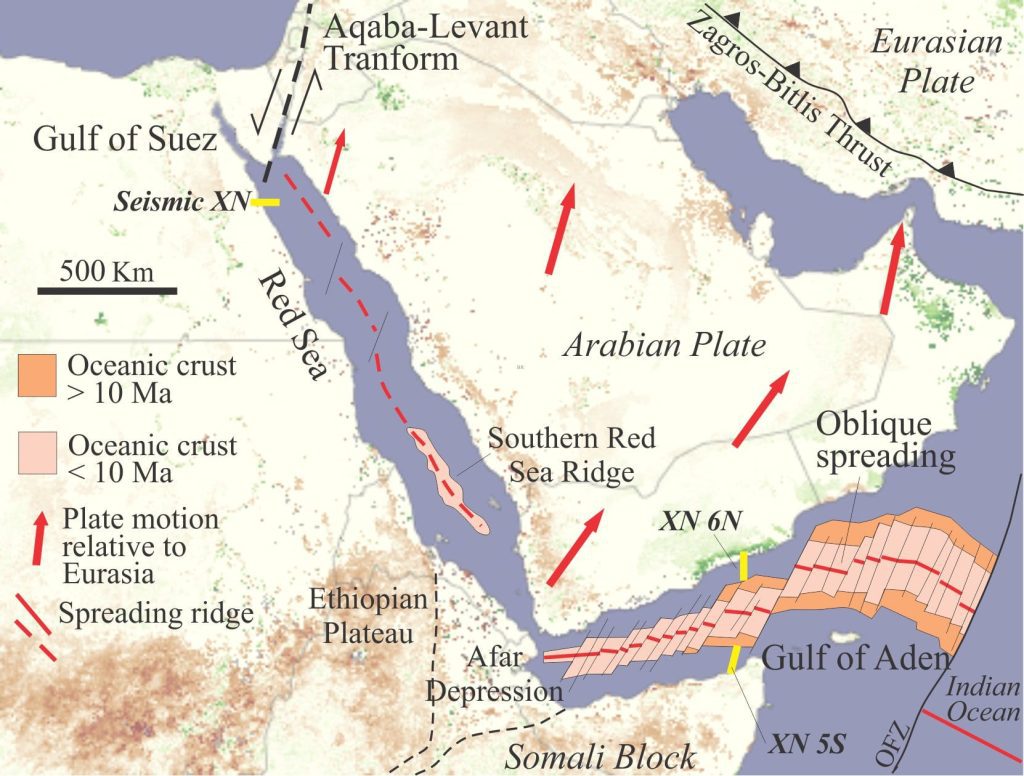
Red Sea and Gulf of Aden rift domains. The Red Sea rift axis merges south with a nascent spreading ridge. Sea floor spreading in Gulf of Aden is diachronous with oceanic crust older than 10 Ma at its eastern limit. The pattern of fracture zones and spreading ridge offsets is consistent with oblique spreading and the relative motion of Arabia. Location of the seismic profiles (below) is indicated. Data from Bosworth, 2015. The base image is from NASA
- Continental rifting in Gulf of Suez with rift-parallel listric faults and associated grabens, that merge with;
- Mature rifting in northern Red Sea, apparently in transition to incipient sea floor spreading that farther south has evolved to
- Full sea floor spreading in southern Red Sea where 85 km of new oceanic crust has been extruded, plus nascent, conjugate passive margins. Crustal thickness along the Red Sea margins (depth to Moho) is about 20 km, thinning from 35-40 km in the plate interiors.
- Sea floor spreading is more advanced in Gulf of Aden and conjugate passive margin successions show some of the characteristics of more mature margins, such as clinoforms.
- Extension in Red Sea has produced troughs as deep as 2300 m.
- Rift shoulders line both sides of the rift system, with elevations locally to 3000 m.
- Northward movement of the Arabian plate (relative to Africa) in concert with rifting, is partly accommodated by left-lateral displacement along the Aqaba-Levant Transform fault (a plate boundary) and underthrusting of the Eurasian plate along the Bitlis-Zagros Thrust.
- Oblique sea floor spreading in Gulf of Aden has produced en echelon, northeast-striking fracture zones that offset the spreading ridge and partition some of the northward movement of Arabia.
Time line of rifting and sea floor spreading (most of this info is from Bosworth and Burke, 2005; Bosworth, 2015; Nonn et al. 2019):
- The beginning of uplift and extension for Red Sea, Gulf of Aden, and EARS was emplacement of a mantle plume beneath Afar about 31 Ma, the eruption of trap basalts, and by 30Ma rhyolites.
- Rifting along future Gulf of Aden began soon after, advancing south towards Eritrea by about 27 Ma. Marine deposits (evaporites, limestones) indicate that the fault-controlled basins had access to the sea.
- Rifting in southern Red Sea began about 24 Ma, accompanied by extensive, rift-parallel dike intrusion.
- Rifting along the entire 4000 km Red Sea – Gulf of Aden system took only 6 million years.
- Sea floor spreading began in eastern Gulf of Aden at 19Ma, propagating to the western Gulf by 10 Ma.
- Isolation of ancestral Red Sea by 14 Ma led to widespread evaporite deposition that continued to about 5 Ma.
- Sea floor spreading began in southern Red Sea at 5 Ma.
- Sea floor spreading from Gulf of Aden to Red Sea is highly diachronous; 19 million years so far.
The architecture of nascent passive margins:
Gulf of Suez and Red Sea
Syn-rift sedimentation began with coarse-grained fluvial and alluvial fan deposits that reflect the developing faulted topography, mostly fluvial-alluvial, with a few marine incursions depositing limestone and evaporites. Rapid subsidence subsequently provided access to sea water and the accommodation for thick marls. The Early syn-rift stage ended about mid-Miocene.
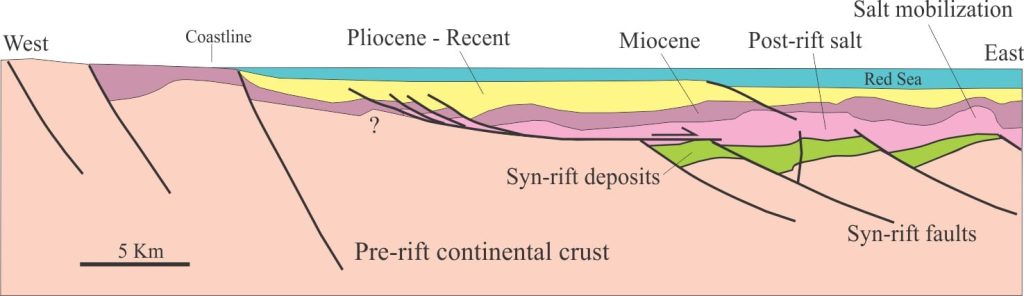
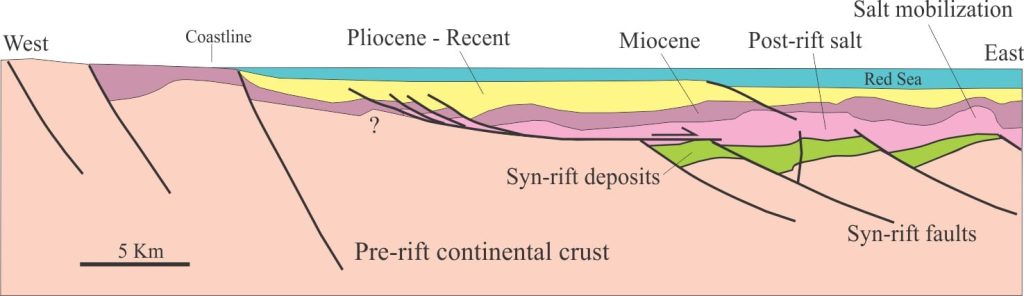
Interpreted profile based on reflection seismic, across the western margin of north Red Sea. Syn-rift deposits occupy half grabens bound by listric faults; some faulting has continued into the overlying post-rift succession. There has been significant mobilization of the post-rift salt, including a large detachment at the base of the salt (mid profile). The post-rift succession will be the foundation of a passive margin once sea floor spreading begins in this sector of Red Sea. From Bosworth and Burke, 2005.
The late rift stage was dominated until the end of the Miocene by deposition of thick halite with thin anhydrite, sandstone, and limestone beds. During the Pliocene and Pleistocene, the salts were buried by shale and sandstone. Post-Miocene deposition was strongly influenced by salt mobilisation, that produced salt walls and domes, some more than 3000 m thick.
A basin-wide unconformity is present beneath Pliocene strata. This has been interpreted as a break-up unconformity that records the transition from continental rifting to sea floor spreading in Red Sea. However, Bosworth and others argue that the unconformity correlates with the late Messinian unconformity in the Mediterranean and therefore is more a reflection of the salinity crisis than rift-drift.
Gulf of Aden
Progression of oblique sea floor spreading in Gulf of Aden, although diachronous, has initiated conjugate margins with detectable (on seismic profiles) transitions from continental to oceanic crust. Depth to the Moho decreases from 35 km inland of the Gulf, to about 20 km in the continental-oceanic crust transition.
Syn-rift grabens bound by listric faults accommodate coarse-grained clastic, carbonate, and halite-anhydrite deposits 1-2 km thick, and in some troughs >3 km thick. Rotation by syndepositional faulting resulted in numerous stratigraphic discordances.
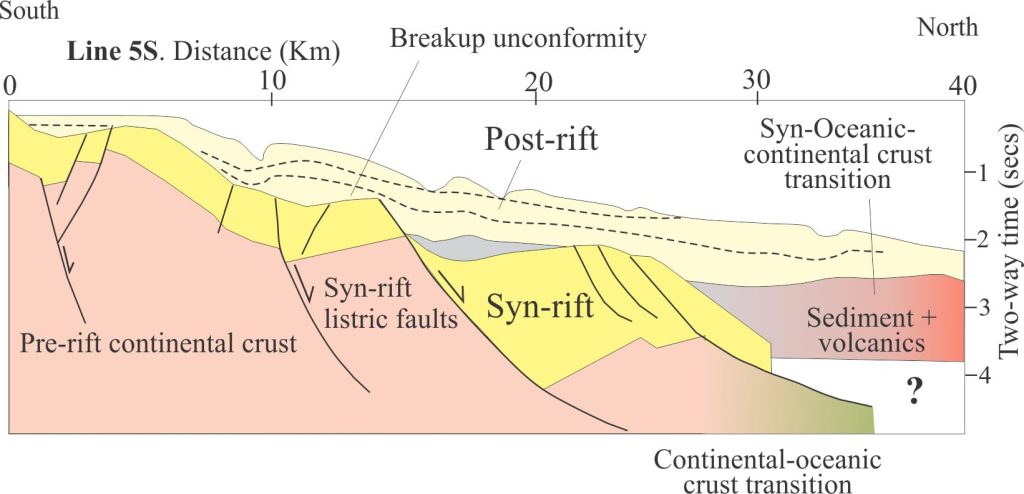
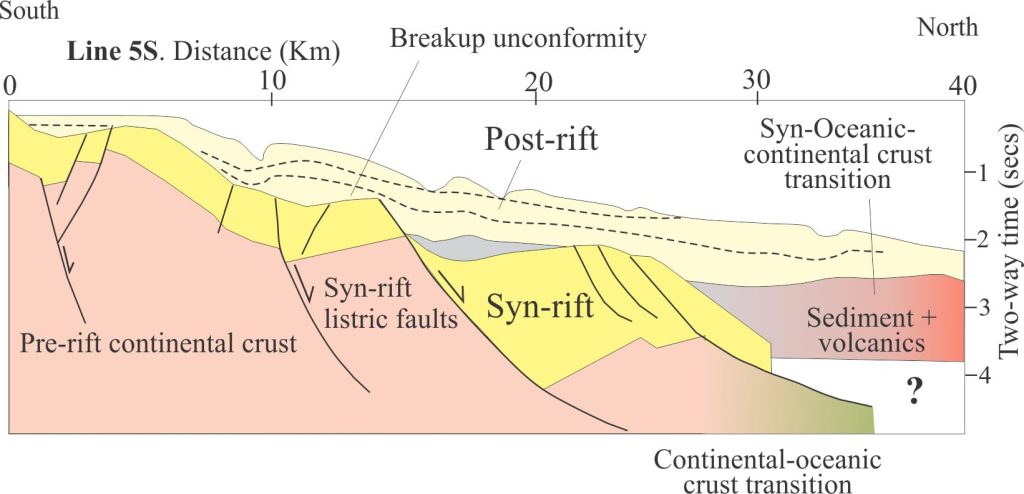
Two profiles across the north (Line 6N) and south (Line 5S) margins of Gulf of Aden interpreted from seismic. Both show the transition from continental crust to crust that is transitional to oceanic. Syn-rift deposits occupy half grabens bound by basin-dipping listric faults, and in turn are unconformably overlain by nascent post-rift, passive margin successions. Prograding clinoforms are well developed in the post-rift succession in Line 6N. Part of the post-rift stage at the basinward end of profile 6N is interpreted as coeval with the continental-oceanic crust transition that probably developed at the beginning of sea floor spreading; the stratigraphic package here includes volcanic accumulations. Modified from Nonn et al. 2019.
Volcanism and intrusion have also played a role in shaping both the syn-rift stage, and the stage where crust transitional between fully continental and fully oceanic formed. In Gulf of Aden, volcanism appears to have been more prominent in the West. The influence of volcanism is apparent in profile 5N where syn-rift and post-rift segmentation of the margin has produced small sub-basins and stratigraphic on lap of associated depositional packages.
The overlying paired passive margin successions extend from a depositional edge near the modern coast, to 2-3 km thick offshore. Some seismic profiles show well defined clinoforms with shoreward onlap and basinward downlap geometries. A mid-Miocene unconformity between the syn-rift and post-rift (drift) sedimentary packages is interpreted as a break-up unconformity. Note that this unconformity is older than that in the Red Sea region (end of Miocene).
A companion post looks at the initiation of continental rifting in the East African Rift System (EARS) and a couple of early but relevant models of rift to passive margin transitions.
Topics in this series
Sedimentary basins: Regions of prolonged subsidence
The rheology of the lithosphere
Isostasy: A lithospheric balancing act
The thermal structure of the lithosphere
Classification of sedimentary basins
Stretching the lithosphere: Rift basins
Basins formed by lithospheric flexure
Accretionary prisms and forearc basins
Basins formed by strike-slip tectonics
Allochthonous terranes – suspect and exotic
Source to sink: Sediment routing systems
Geohistory 1: Accounting for basin subsidence
Geohistory 2: Backstripping tectonic subsidence
























